Invited Speakers
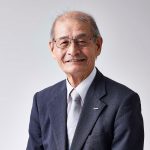
Akira Yoshino
Honorary Fellow
Asahi Kasei Corporation
Japan
Dr. Akira Yoshino is Honorary Fellow of Asahi Kasei Corporation, Director of Global Zero Emission Research Center of National Institute of Advanced Industrial Science and Technology (AIST), and President of the Lithium Ion Battery Technology and Evaluation Center (LIBTEC). He began research on rechargeable batteries in 1981, and in 1983 fabricated a prototype rechargeable battery using lithium cobalt oxide as cathode and polyacetylene as anode. He switched to carbonaceous material for the anode and in 1985 fabricated and received the basic patent for the first prototype of the LIB.
He developed the aluminum foil current collector, which formed a passivation layer to enable high cell voltage at low cost, and developed the functional separator membrane and the use of a positive temperature coefficient (PTC) device for additional safety. He also conceived of the LIB’s coil-wound structure to provide large electrode surface area and enable high current discharge despite the low conductivity of the organic electrolyte.
Dr. Yoshino has received numerous national and international awards, including the IEEE Medal for Environmental Safety Technologies in 2012, the Global Energy Prize in 2013, and the European Inventor Award and the Nobel Prize in Chemistry in 2019. He received his BS and MS from Kyoto University and his EngD from Osaka University.
The lithium-ion battery (LIB) is a small and lightweight rechargeable battery which greatly contributed to the advent of the mobile IT society. For over 25 years in consumer electronics applications, the LIB’s performance and reliability have been improved while its cost has been reduced. Currently the LIB is at a turning point in automotive applications. This will not simply be electrification of vehicle drive systems, but integration with the latest technologies such as IoT, AI, and 5G. This is expected to usher in a major social transformation in terms of the future of mobility.
What will the electrification of vehicles be like from 2025 onward, and how will the LIB contribute to a sustainable society? We often hear the buzzword “CASE” regarding the future of mobility. This is an important concept. “C” stands for connected, meaning vehicles will be connected to the internet, which is the concept of IoT. “A” stands for autonomous, meaning vehicles will drive without humans operating them, which is a kind of AI technology. “S” stands for shared, meaning vehicles that are not individually owned. “E” stands for electric, meaning vehicle drive systems will be electrified. These four aspects of the CASE concept are symbolic of the future of mobility.
The negative legacies of the industrial revolution, global environmental issues require a fundamental solution. In combination with new technologies such as AI, IoT, and 5G, the LIB can enable a truly sustainable society.
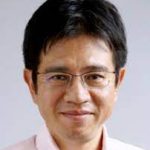
Atsuo Yamada
Professor
The University of Tokyo
Japan
Atsuo Yamada has unique career covering both academic and industrial research. After serving as a laboratory head of Sony Research Center, he was immediately appointed as an associate professor at Tokyo Institute of Technology in 2002, a full professor of the University of Tokyo in 2009. During the period, he joined the John. B. Goodenough’s lab. in University of Texas at Austin as a visiting scholar for one year, and was called for sabbatical stay from University of Bordeaux as an invited professor to enhance research communication with Dr. Claude Delmas in ICMCB/CNRS.
His diverse research works on battery materials, particularly recognized for sophisticated approaches for structure-property relationships, include very early-stage exploration/optimization of LFP and more recently, identification and understanding of several functional electrolytes. He holds 90 patents, published 25 chapters and well over 270 refereed journal papers with total citation exceeding 30,000, delivering 150 plenary/keynote/invited presentations, and ranked as a Highly Cited Researcher by Clarivate Analytics, and now serving for the scientific advisory board of Advanced Energy Materials.
Among his many honors, Atsuo has been awarded the Spriggs Award and the Purdy Award from ACerS, the Scientific Achievement Award from ECS Japan, and IBA Research Award from International Battery Association, and Battery Division Research Award from the Electrochemical Society.
In any electrochemical system, electrode potential is the central variable that regulates the driving force of redox reactions. However, quantitative understanding of the electrolyte dependence has been limited to the classic Debye-Hückel theory that approximates the Coulombic interactions in the electrolyte under the dilute limit conditions. Therefore, accurate expression of electrode potential for practical electrochemical systems has been a holy grail of electrochemistry research for over a century. Here we show that the ‘liquid Madelung potential’ based on the conventional explicit treatment of solid- state Coulombic interactions enables quantitatively accurate expression of the electrode potential, with the Madelung shift obtained from molecular dynamics reproducing a hitherto-unexplained huge experimental shift for the several battery electrodes. Furthermore, incorporating the effects of entropy and polarization dynamics could polish the theoretical model to perfectly fit the experimental data. Thus, a long-awaited method for the description of the electrode potential in any electrochemical system is now available.
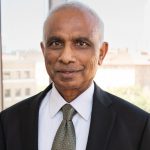
Arumugam Manthiram
George T. and Gladys H. Abell Endowed Chair of Engineering
University of Texas at Austin
United States
Arumugam Manthiram is currently the George T. and Gladys H. Abell Endowed Chair of Engineering at the University of Texas at Austin (UT-Austin). He served as the Director of the Texas Materials Institute and the Materials Science and Engineering Program at UT-Austin for 11 years during 2011 – 2022. He received his Ph.D. degree in chemistry in 1980 from Indian Institute of Technology Madras. After working as a lecturer in chemistry at the Madurai Kamaraj University for 4 years and as a postdoctoral fellow both at the University of Oxford and at UT-Austin, he became a faculty at UT-Austin in 1991. His research is focused on new materials development for batteries and a fundamental understanding of the intricacies involved. He has authored 980 journal articles with 117,000 citations and an h-index of 167. He has mentored ~ 300 students and postdoctoral researchers, including the graduation of 72 Ph.D. students. He founded two startup companies, ActaCell Energy Systems in 2007 and TexPower EV Technologies in 2019.
He is an elected Fellow of the U.S. National Academy of Inventors, Materials Research Society, Electrochemical Society, American Ceramic Society, Royal Society of Chemistry, and American Association for the Advancement of Science. He is an elected Academician of the World Academy of Ceramics. He received the Battery Division Research Award in 2014, Henry B. Linford Award for Distinguished Teaching in 2020, Battery Division Technology Award in 2021, and the Inaugural John B. Goodenough Award in 2023, all from the Electrochemical Society. He received the Distinguished Alumnus Award of the Indian Institute of Technology Madras in 2015, Billy and Claude R. Hocott Distinguished Centennial Engineering Research Award in 2016, and International Battery Materials Association (IBA) Research Award in 2020. He delivered the 2019 Chemistry Nobel Prize Lecture in Stockholm on behalf of Professor John Goodenough.
With an aim to enhance the energy density while lowering the cost, layered oxide cathodes with high nickel contents with low or no cobalt have come to the forefront. However, increasing the Ni contents above about 80% is met with severe challenges, such as inferior cycle and thermal stabilities. These challenges are linked largely to the instability of highly oxidized Ni4+ in contact with the electrolyte. The instability leads to aggressive surface reactivity, which results in a reduction of Ni4+ to Ni2+ on the surface, loss of oxygen, formation of resistive rock-salt phases, formation of cracks, loss of active lithium, Ni dissolution and migration to the anode, and consequent capacity fade during extended cycling. The reactivity also leads to thermal instability, gas evolution, and safety concerns.
To overcome the challenges, generally doping of high-Ni cathodes with various dopants is pursued. However, no clear understanding is available in the literature on which dopant does what. Doping is generally carried out randomly in the literature by a trial and error process. This presentation will provide a systematic investigation of doping LiNiO2 with various dopants, such as Co, Mn, Al, Mg, Ti, Nb etc. The effect of dopants on capacity, cycle life, thermal stability, and gas evolution will be presented, providing the underlying mechanisms involved in the benefits offered by each dopant. The surface reactivity depends both on the surface characteristics of high-Ni cathodes and the electrolyte. This presentation will then focus on the development of cathodes with a robust surface as well as more stable electrolytes to reduce the challenges of surface reactivity. The ability to achieve long cycle life with high capacity, while reducing the amount of gas evolution and increasing the charging rates, by tuning the cathode and electrolyte compositions will be presented. Also, the need to pay attention towards two-way crossover in cells employing lithium-metal or silicon anode will be emphasized. Finally, the fundamental understanding developed with the use of advanced analytical techniques, such as in-situ XRD, SEM, XPS, and TOF-SIMS will be discussed.
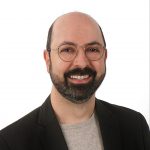
Babak Shalchi, PhD.
Research Scientist
CanmetMATERIALS
Canada
Dr. Babak Shalchi obtained his PhD from the University of Alberta in 2011, where he focused on novel nano-structured hydrogen storage materials, marking the beginning of his journey into the field of sustainable energy solutions. Following his postdoctoral fellowship at NINT (now Nanotechnology Research Centre), where he explored supercapacitors and lithium-ion batteries, Dr. Shalchi joined CanmetMATERIALS lab (Natural Resources Canada) in Hamilton as a Research Scientist.
At CanmetMATERIALS, Dr. Shalchi serves as the lead Transmission Electron Microscopy (TEM) scientist, playing a pivotal role in advancing characterization techniques in materials science. His expertise in TEM has been instrumental in elucidating complex structures and phenomena within engineering alloys and energy materials, contributing to the development of lighter, stronger alloys and more efficient energy materials. With over 150 journal articles he has made significant contributions to various aspects of materials science, including alloy design for steels and aluminum alloys, additive manufacturing, and welding metallurgy.
In addition to his role at CanmetMATERIALS, Dr. Shalchi holds adjunct professorships at the University of Waterloo and the University of New Brunswick, where he fosters interdisciplinary collaboration and contributes to research across diverse domains. Through his collaborative approach and prolific publication record, Dr. Shalchi continues to contribute to the advancement of materials science, with a particular focus on developing sustainable energy solution.
Sustainability for lithium-ion batteries (LIBs) is driven by the necessity to reduce reliance on cobalt, a critical mineral entangled with environmental and ethical concerns, while also addressing the challenge of graphite resource depletion. This presentation highlights advancements in photon- and electron-based characterization strategies aimed at enhancing LIBs ecological balance.
First, we explore the enrichment of polycrystalline nickel-rich cathode structures with tungsten (W), unveiling the role of W in improving their electrochemical performance and structural stability. Employing advanced synchrotron-based characterization and electron microscopy techniques, discover various W-rich phases in both amorphous and crystalline forms with heterogeneity distribution, contributing to better cycling performance.
Next, we showcase the innovative application of W as a dopant in all-dry single crystal cathodes, highlighting the advancement in using single crystal and attempt to control over cathode structure and composition by this approach. High-resolution electron microscopy explains structural details about the distribution of transmission metals and introduced W elements, illustrating their potential to revolutionize LIB performance by enhancing energy density and cycling stability.
Furthermore, we present a comprehensive study on the recycling and purifying graphite for anodes from black mass, a byproduct of LIB recycling processes. Employing advanced electron microscopy techniques along with other chemical and electrochemical analyses, we pinpoint key parameters critical for evaluating the cycling performance of recycled graphite, paving the way for the development of sustainable anode materials with minimal environmental impact.
These advancements could help facilitating the widespread adoption of clean energy technologies and reducing carbon emissions while diminishing economic dependency on imported virgin materials.
Keywords: Electron microscopy, Sustainability, Cathode structures, Graphite reclamation, Tungsten doping, Recycling and Lithium-ion batteries.
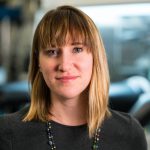
Betar Gallant
Professor
MIT
USA
Betar M. Gallant is an Associate Professor and the Class of ‘22 Career Development Professor in the Department of Mechanical Engineering at MIT, where she leads the Energy and Carbon Conversion Laboratory. She obtained her SB, SM, and PhD degrees from this department. Following her PhD, Dr. Gallant was a Kavli Nanoscience Institute Postdoctoral Fellow at Caltech. Her research group at MIT focuses on advanced battery chemistries and materials for high-energy primary and rechargeable batteries, including fluorinated cathode conversion reactions and lithium and calcium metal anodes and their interfaces. Her group is also leading research into CO2 capture and its integration with direct electrochemical conversion in the captured state. She is the recipient of multiple awards including an MIT Bose Fellow Award, Army Research Office Young Investigator Award, Scialog Fellow in Energy Storage, Scialog Fellow in Negative Emissions Science, National Science Foundation CAREER Award, The Electrochemical Society (ECS) Battery Division Early Career Award, an ECS-Toyota Young Investigator Award, and the Ruth and Joel Spira Award for Distinguished Teaching at MIT.
The lithium (Li) metal anode promises significantly higher capacity than graphite and is central to strategies to develop advanced Li-ion batteries with improved energy, lifetime and performance. Yet, the Coulombic efficiency (CE) of Li anodes in liquid electrolytes still falls well below the >99.9% targeted for electric vehicles. Efficiency and capacity loss arise from uncontrolled reactivity at the solid electrolyte interphase (SEI) and its resulting physicochemical, ionic and electronic properties, which couple to inhomogeneous plating/stripping, SEI breakage, electrolyte infiltration and additional consumption, and ultimately to loss of active Li inventory as stranded Li0 or SEI Li+. Despite empirical insights into electrolyte design guidelines, quantitative understanding of SEI functionality is underdeveloped, hindering attempts to control and improve its properties through tailoring of its materials chemistry.
This talk will discuss our ongoing efforts to improve understanding of phase-specific functionality in the SEI. Our work aims to advance quantitative techniques to yield insights into SEI phases and the hidden interplays between their chemistry, properties and function, with an objective of identifying design principles to minimize active Li inventory loss. We developed approaches to isolate and synthesize SEI-relevant ionic and organic phases, including lithium oxide (Li2O), lithium fluoride (LiF) and recently lithium carbonate (Li2CO3), at representative nanometer-scale thicknesses directly on Li metal. These interfaces are interrogated via targeted electrochemical techniques to reveal their Li+ conductivity, providing a framework to assess which phases bolster, or hinder, ion transport in the SEI. In this talk, we examine in detail the role of Li2CO3, a pervasive yet metastable SEI component that has long been asserted to improve CE in certain electrolytes. By synthesizing model Li2CO3 interphases, we measure the ionic conductivity, probe reaction pathways in contact with specific electrolyte constituents, and interpret its properties against other key ionic SEI phases. We further examine the role of carbonate functionality in the native SEI, revealing a mechanism by which Li2CO3 and related phases can improve CE. Finally, we look holistically across model phases studied to date to infer future objectives for improving SEI composition.
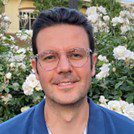
Bryan McCloskey
Professor of Chemical Engineering in the Department of Chemical and Biomolecular Engineering
University of California, Berkeley
USA
Bryan McCloskey is the Department Chair and Warren & Katharine Schlinger Distinguished Professor in Chemical Engineering in the Department of Chemical and Biomolecular Engineering at the University of California, Berkeley. He also holds a joint appointment as a Faculty Engineer in the Energy Storage and Distributed Resources Division at Lawrence Berkeley National Laboratory. His laboratory explores numerous applications of electrochemistry to energy sustainability, conversion, and storage. Current projects focus on electrochemical regeneration of alkaline sorbents for direct air CO2 capture, elucidating the fundamental electrochemistry of metal-air batteries, and understanding a variety of challenges facing Li-ion batteries, including high voltage cathode stability, advanced cathode material development (Ni-rich and Li- rich NMC oxides and Li-excess disordered rocksalt materials), extreme fast charging, and low temperature and high transference number electrolyte formulations. He has co-authored more than 150 articles and has won numerous awards for his research, including The Electrochemical Society Charles Tobias Award, The International Society of Electrochemistry Tajima Prize, and the VW/BASF Science Award- Electrochemistry. More information about the McCloskey Lab can be found at the Lab’s website: www.mccloskeylab.com.
Li-ion battery fast charge is limited due to challenges posed by lithium plating on the graphite anode, whereby the large overvoltage necessary to drive high Li+ insertion rates instead results in favorable thermodynamic conditions for Li metal deposition on the graphite surface. Li plating is difficult to detect, particularly in small quantities, and results in safety risks and capacity fade due to lithium metal’s high reactivity with conventional electrolytes. Here, we present our efforts to develop both chemical and electrochemical methods to precisely quantify Li plating and detect the state-of-charge (SOC) onset of lithium plating on graphite electrodes during constant current fast charging. We will discuss titration mass spectrometry (TiMS), a highly sensitive (~20 nmol resolution, or 0.5 mAh of plated Li) chemical analysis where gases evolved from harvested graphite electrodes immersed in acid are used to quantify various solid electrolyte interphase species, including electrically isolated (inactive) Li metal.1 TiMS is then used to determine the precise onset of Li plating and to distinguish between the various capacity fade mechanisms that arise during fast charge.2 We then will discuss the use of simple electrochemical cycling techniques to quantify irreversible Li plating in Li|Graphite half-cells as a function of energy density (electrode thickness), charge rate, temperature, and SOC.3 Similar methods are developed to quantify in-situ Li plating for commercially relevant Graphite|LiNi0.5Mn0.3Co0.2O2 (NMC) cells. In combination, all of these techniques provide a highly accurate measure of the onset of Li plating and quantitative insight into capacity losses during fast charging.
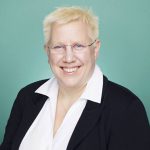
Celina Mikolajczak
Mechanical Engineer
Lyten
USA
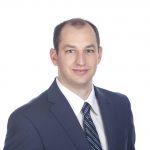
Chris Burns
CEO
NOVONIX
Canada
Chief Executive Officer
• Previously led all company divisions including NOVONIX Battery Technology Solutions and NOVONIX Anode Materials
• Co-developed the breakthrough Ultra High Precision Coulometry (UHPC) technology with Professor Jeff Dahn
• Co-founded NOVONIX Battery Technology Solutions in Canada in 2013
• Former Senior Research Engineer with Tesla
• As Tesla’s first technical hire in Canada in 2015, Dr. Burns led projects in materials and battery characterization with the goals of selecting EV and ESS battery chemistry and materials
• Dr. Burns holds multiple patents related to Li-ion batteries and is a co-author on peer reviewed journal articles that have been cited over 4,000 times
• Received his PhD and M.Phys. in Physics from Dalhousie University in Halifax, Nova Scotia, and his BS in Physics from St. Francis Xavier University in Nova Scotia
Innovation is the lifeblood of progress, but navigating the complex landscape of research, development, and commercialization requires vision, strategy, and adaptability. In this presentation, Dr. Chris Burns will share his insights garnered from his journey leading NOVONIX, a company of 200+ people and one of the most innovative in the battery sector.
At the helm of NOVONIX since its founding in 2013, Dr. Burns has led NOVONIX on a mission to develop innovative, sustainable technologies, such as their world-class Ultra-High Precision Coulometry systems and high-performance anode and cathode materials to service the electric vehicle and energy storage industries. Central to this endeavor has been the assembly of a world-class R&D team, breakthroughs in battery testing technology, the development of high-performance battery materials, and much more. But innovation isn't just about generating ideas — it's about identifying when those ideas are ripe for the next stage of development, like the company recently did with its all-dry, zero-waste cathode synthesis process. Dr. Burns will unravel the strategic decisions behind transitioning innovative concepts from laboratory to market. Delving into critical junctures where readiness meets opportunity, he will reveal the delicate balance between scientific rigor and commercial viability.
Dr. Burns will also explore the art of navigating the intersection between scientific discovery and business acumen. While scientific excellence forms the cornerstone of NOVONIX’s endeavors, the company has embraced the imperative of elevating innovations to a commercial level, ensuring their transformative potential reaches the widest possible audience.
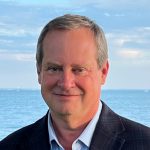
Christopher Johnson
Senior Chemist & Argonne Distinguished fellow
Argonne National Laboratory
USA
Dr. Christopher S. Johnson is currently a Senior Chemist and an Argonne Distinguished Fellow at Argonne National Laboratory, specializing in the research & development of battery materials and battery systems with 32 years of experience. He is known worldwide for his development of state-of-art lithium-ion battery cathode materials, and recently, Si anodes, and sodium-ion batteries. He earned his Ph.D. in Chemistry from Northwestern University following undergraduate studies and a B.S. Chemistry at University of North Carolina at Chapel Hill. He retains a joint position as Senior Chemist At-Large and Lab Innovation Fellow at the University of Chicago. He has published over 150 publications (h-index 68), and 35 issued US patents. He has received the Battery Research Award from the International Battery Association in 2006, and a R&D 100 Award in 2009 for the Commercialization of the NMC Cathode for Lithium-ion Batteries. He is the 2018 recipient of the University of Chicago Argonne Distinguished Scientist Award, and is a Fellow of the Electrochemical Society.
The improvement in electrode performance properties of layered sodium transition metal oxide cathodes from baseline NaNi1/3Mn1/3Fe1/3O2 (NMF) to advanced materials will be reported. Through a combination of layer stacking P-type perturbations, precise Na/Mn ratios, selected dopings and intergrowths, a new class of stable high SOC materials with layered orientation relationships have been produced. These advanced cathodes are slated towards increasing sodium-ion battery energy densities to >160 Wh/kg in single layer pouch cells.
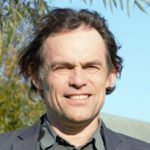
Christian Masquelier
Professor
Université de Picardie Jules Verne
France
Christian Masquelier joined Université de Picardie Jules Verne, Amiens, France, as a Full Professor of Chemistry. He has been working for >30 years on the crystal chemistry of sodium ion conductors and positive electrode materials for Li-ion and Na-ion batteries, in particular on operando X-ray or neutron diffraction of phosphate-based positive electrodes. He is the co-author of ~170 publications and 21 international patents in this field. He is a Senior Member of the Institut Universitaire de France, presently Co-Director of the ALISTORE European Research Institute and Deputy Director of the Laboratoire de Réactivité et de Chimie des Solides (LRCS) in Amiens, France
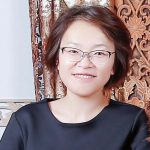
Chunmei Ban
Associate Professor
University of Colorado Boulder
USA
Chunmei Ban is an Associate Professor at the Paul M. Rady Department of Mechanical Engineering and affiliated with Materials Science and Engineering Program at University of Colorado Boulder, Boulder, CO. Prior to 2019, Ban was a Senior Scientist (V) in the Chemistry and Nanoscience Center at National Renewable Energy Laboratory (NREL), Golden, CO, has led DOE-awarded projects in intermetallic anodes, high-energy cathodes, and direct recycling process for battery materials. Ban received a Bachelor’s and Master’s degree from the Department of Chemical Engineering from Tianjin University, China and holds a PhD in Chemistry from the State University of New York at Binghamton, supervised by Prof. M Stanley Whittingham, a 2019 Nobel Laureate in Chemistry. Her current research interests lie on comprehending the dynamic properties of the electrode-electrolyte interface during the electrochemical processes, and leveraging this understanding to advance the development of next-generation electrode and electrolyte materials for energy storage applications.
Sodium-based beyond-lithium batteries offer promising and sustainable alternatives to commercially available lithium-ion batteries, thanks to the low-cost and high abundance of sodium sources ($2433 per ton, constituting 2.4% of the earth’s crust). Despite having a larger mass than lithium, sodium-ion batteries have the potential to achieve a gravimetric energy density reaching 170 Wh/kg at the cell level, owing to a rich selection of intercalation cathodes. However, to achieve highly reversible, long-lasting cycling performance, it is essential to facilitate the formation of robust electrode-electrolyte interfaces in shielding the electrodes from undesirable side reactions. Therefore, the pursuit of low-cost and high-performance electrolytes is crucial to unlocking the full potential of sodium-ion batteries. Here, this presentation will discuss our strategies in developing electrolytes for sodium-ion batteries. Rather than relying on conventional electrolyte salts, we focus on the exploration of fluorine-free electrolytes and their impact on the formation of the electrode-electrolyte interface. This approach offers a promising alternative to fluorine-containing electrolytes by harnessing safe, abundant and cost-effective materials.
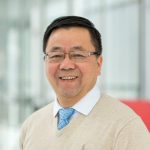
Chunsheng Wang
Department of Chemical and Biomolecular Engineering
University of Maryland
USA
Dr. Chunsheng Wang is a Robert Franklin and Frances Riggs Wright Distinguished Chair Professor in Chemical and Biomolecular Engineering at the University of Maryland. He is an associate editor of ACS Applied Energy Materials, UMD Director of the UMD-ARL Center for Research in Extreme Battery, and a Fellow of the Electrochemical Society. His research focuses on rechargeable batteries and fuel cells. He has published more than 350 papers. His work has been cited more than 73940 times with an H-index of 143. He has been listed as a Highly Cited Researcher since 2018 by Clarivate and received the 2021 Battery Division Research Award from The Electrochemical Society.
The energy density, safety, and cycle life of rechargeable batteries are critical for electric vehicles (EV), electric aviation, and renewable energy storage. However, current Li-ion batteries still cannot simultaneously meet all these requirements. We reported electrolyte design principles that enable high-energy Li-ion and Li metal batteries to achieve a long cycle life with a wider operation temperature.
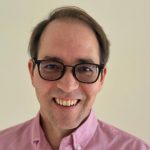
Daniel Friebel
Director of R&D
Natron Energy
USA
Daniel Friebel is the Director of R&D at Natron Energy, where he leads key discoveries related to Natron’s unique Prussian Blue cell chemistry and supports the scale-up of active materials manufacturing to metric-ton batches. Daniel joined Natron in 2018 and has led the R&D team since 2020. As a German native, Daniel obtained his Ph.D. in Chemistry at University of Bonn in 2007. After that, he served for 9 years as scientist and co-PI at SLAC National Accelerator Laboratory, where he led pioneering in situ and operando x-ray spectroscopy studies in fuel cell catalysis, water electrolysis and electrochemical carbon dioxide reduction. Daniel has co-authored 30+ peer-reviewed publications on electrocatalysis, x-ray spectroscopy and scanning tunneling microscopy.
Prussian Blue Analog (PBA) materials have been widely investigated as cathode active materials for Na-ion and K-ion cells. Their unique ability to undergo alkali ion intercalation/deintercalation reactions with only minimal structural changes to their host lattice can result in extremely long cycle life through deep discharge cycles. Examples of high-voltage Na-ion and K-ion cells with PBA cathodes have been demonstrated in which the anode material is hard carbon and graphite, respectively. However, achieving a stable anode SEI remains an unsatisfactorily addressed challenge in these high-voltage systems. Natron Energy has developed and commercialized the first Na-ion battery that is entirely based on PBA active materials in both positive and negative electrodes. We invented a PBA negative electrode material based on hexacyanomanganate(II/I) redox at a low potential near 1.9 V vs. Na/Na+. When sodium manganese hexacyanomanganate negative electrodes are paired with positive electrodes based on hexacyanoferrate PBAs, full cell voltages up to 2V can be achieved. While the open structure of PBAs and low voltage limit energy density, these cells are capable of extremely fast full charge (up to 20C) and deep discharge (up to 60C), pose no risk of thermal runaway, and achieve exceptionally long service life with tens of thousands of cycles [1]. Insight into Natron’s progress in scaling up active materials production and mass manufacturing of cells will be given, and the unique high-rate deep-discharge capabilities will be discussed in the context of commercial applications where Na-ion batteries can close important gaps that are not addressed with Li-ion batteries, such as uninterruptible power supplies, demand charge management, and support for electric vehicle fast charging.
[1] "Assessment of the first commercial Prussian blue based sodium-ion battery," He, Minglong, et al, J. Power Sources 548 (2022) 232036.
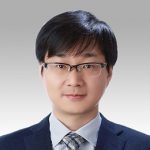
Dong-Hwa Seo
Professor of Materials Science and Engineering
Korea Advanced Institute of Science and Technology (KAIST)
South Korea
Dong-Hwa Seo is an associate professor of materials science and engineering at Korea Advanced Institute of Science and Technology (KAIST), where he completed his PhD in 2011. He was an assistant professor of school of energy and chemical engineering at Ulsan National Institute of Science and Technology (UNIST) from 2019 to 2023. He worked at Samsung Research America as a research engineer (2017-2019), University of California, Berkeley as a research specialist (2016-2017), and Massachusetts Institute of Technology (MIT) as a postdoctoral researcher. His research is mainly focused on understanding the reaction mechanism of the battery materials and their interfaces at the atomic scale using first-principles calculation and developing high-performance battery materials based on the in-depth understanding. His published works in this field have been cited more than 17,000 times. He was a recipient of Early Career Award from the International Battery Materials Association (IBA) in 2018 and selected as Highly Cited Researchers in 2022 from Clarivate Analytics.
The shift towards electric vehicles and large-scale energy storage systems necessitates cost-effective and abundant alternatives for the commonly used Co/Ni-based cathodes (like LiNi0.6Mn0.2Co0.2O2) in Li-ion batteries (LIBs). Manganese-based disordered rock-salts (Mn-DRXs) have shown a potential to exceed the performance of traditional cathodes at a reduced cost, achieving more than 900 Wh/kg-AM (active material), but this has only been proven in cell designs that are not commercially viable. These designs use diluted electrode films (about 70 wt% AM) with excess carbon and binder. In this work, we reveal that the failures of Mn-DRXs in AM-concentrated electrodes stem from their low electrical conductivity and the collapse of their electrical network with volume change during charge and discharge. We overcome these challenges through the engineering of electrical percolation, showcasing highly concentrated electrode films of Mn-DRX cathodes (approximately 96 wt% AM) and achieving the highest reported energy density at the electrode level (around 1050 Wh/kg-cathode). This research also emphasizes the balancing effect of manganese content on the electrical conductivity and volume change of Mn-DRXs, pushing forward the development of Co/Ni-free LIB technology.
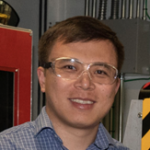
Enyuan Hu
Chemist & Principal Investigator (PI)
Brookhaven National Laboratory
New York
Dr. Enyuan Hu received his Ph.D. from Stony Brook University and his bachelor's degree from Southeast University in China. He is currently a chemist and a principal investigator (PI) at the Chemistry Division of Brookhaven National Laboratory. He works as PI/co-PI on several DOE-funded projects including the Battery500 Consortium. His research areas include lithium metal, lithium sulfur, sodium-ion, and solid-state batteries and he pioneered the use of synchrotron x-ray total scattering to characterize interphases in lithium batteries and the use of neutron total scattering to study the oxygen redox reaction in battery cathode materials. He has been recognized as a Clarivate Highly Cited Researcher in both 2022 and 2023 and received the International Battery Material Association (IBA) Early Career Award in 2023.
We have identified several new lithium battery electrolyte solvents and additives with various extents of success. The isoxazole-based electrolyte has very high ionic conductivity, but its application is limited by its electrochemical stability. Fluorinated sulfonate offers significantly improved stability for the lithium metal anode compared to conventional sulfone solvents. When combined with its intrinsic cathode stability, a 2,2,2-trifluoroethyl mesylate-based, single salt, single solvent electrolyte enables stable cycling of high-loading NMC811 in low N/P ratio lithium metal battery coin cells. Cesium nitrate has been identified as capable of forming protective interphases on both the cathode and anode. Unexpectedly, the lithium metal anode interphase is dominated by cesium bis(fluorosulfonyl) imide (CsFSI) instead of LiF. When used as additive in electrolyte with lithium bis(fluorosulfonyl) imide (LiFSI) as salt and 1,2-dimethoxyethane (DME) as solvent, stable cycling at fast charge/discharge rate can be enabled for lithium metal batteries. More recently, a fluorinated linear carbonate has been identified as a promising solvent for lithium-ion batteries under extreme conditions including low and high temperature operation, fast charging, and high voltage.
In parallel with finding new solvents and additives, we gain more understanding of the interphases through the synchrotron x-ray total scattering technique we previously developed for interphase characterization. LiH, a component previously found to be prevalent in lithium metal interphase, is now understood to have complicated origins, including native surface film and solvent decomposition. Solvent oxidation by cathode seems to be a very influential factor for some electrolyte systems. Through qualifying the Li2O and LiF content and working closely with theoretical group, it is understood that Li2O, an anion decomposition product, strongly absorbs the solvent and catalyzes its decomposition, playing a pivotal role in transitioning from an anion-derived interphase to a solvent-derived one in fluorinated ether electrolyte.
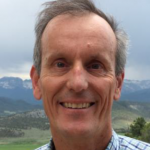
Eric Darcy
NASA
USA
Eric C. Darcy, Ph.D , has spent his 37 year career at NASA in the areas of battery design, verification, and safety assessments for the rigors of manned spacecraft applications. As Battery Technical Discipline Lead at NASA JSC, his main objective has been the development of safe, while high performing, battery systems with a deep focus on understanding, preventing, and mitigating latent defects that could lead to catastrophic cell internal short circuits. With National Renewable Energy Laboratory (NREL) colleagues, he is co inventor of the
patented On demand Internal Short Circuit Device that has provided significant design insights into the cell response during thermal runaway (TR), enabled valid battery TR propagation assessment, and received the prestigious R&D100 award in 2016 and Runner up NASA Invention of 2017.
He’s also co inventor of the Fractional Thermal Runaway Calorimeter for discerning distribution
of TR heat responses of cell designs. This received NASA’s Invention of the Year for 2023. He has led NASA’s design and test efforts for providing a path for developing safe, high performing
Li ion spacecraft batteries using small commercial. He’s published battery design guidelines for
achieving passive TR propagation resistance (PPR). He teaches a Li ion battery safety course
with emphasis on design features and verification measures for achieving PPR battery design s .
Lifetime Achievement Award recipient by NAATBatt in Feb, 2023.
Cylindrical cells achieving up to 250 Wh/kg with Al coated plastic cathode current collectors (PCC) have demonstrated consistent tolerance to sharp and blunt nail penetrations at all states of charge. Imagery and tomography during penetration along with nail force measurements indicate that the PCC makes the jellyrolls more flexible and less penetration force is required than with jellyrolls from control cells using metal foil CCs. Radiography and cell teardowns indicate that cathode active material at the nail interface delaminates from the vaporizing current collector and becomes electrically stranded from the rest of the jellyroll. However, our custom cell designs achieving >275 Wh/kg in an 21700 format consistently overwhelm the PCC and response with immediate thermal runaway. This includes trials with thermally stable ceramic separators and lower cathode binder content. And tests of the 275 Wh/kg cells at 70% SOC also show no nail tolerance. We are currently investigating the role of jellyroll winding tension as a controlling key factor.
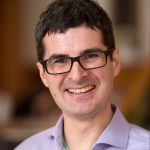
Eric McCalla
Assistant Professor
McGill University
Canada
Eric McCalla completed his PhD at Dalhousie in 2013 and a post-doc at the Collège-de-France in 2015, both of which focused on battery materials research. Following this, he completed a post-doc at the University of Minnesota looking at electronic and magnetic properties of complex oxides. Since 2018, he has been an assistant professor in the Chemistry department at McGill University. His group focusses on developing and applying high-throughput methods to accelerate the development and understanding of advanced battery materials. He is the co-author of 56 published articles (22 as the principal investigator) and 5 patents.
Advanced battery materials for next-generation technologies involve complex chemistries (at least pseudo-quaternary). These materials typically lie in systems where only a handful of compositions have been explored. Furthermore, in cases where further substitutions may prove useful, only a few substituents have been considered in traditional battery development. To fully understand the impact of composition and structure upon the battery properties, thousands of samples are required. Herein, a suite of high-throughput (HTP) methods (a maximum total throughput of 892 samples/week) developed and utilized in the McCalla lab is presented. HTP experiments include X-ray diffraction, electrochemistry (to yield important metrics such as energy density, cycling stability, and electrochemical stability window), electrochemical impedance spectroscopy (to determine ionic conductivity and stability against metallic anodes), and DC conductivity measurements. These methods, all in high-throughput, permit the systematic design of cathodes, anodes and solid electrolytes for both Li- and Na-ion batteries.
This presentation will then demonstrate progress made in developing various components of advanced batteries using our HTP experiments, both showing improved performance and highlighting new understanding about these materials. The Li- and Na-ion battery components studied include cathodes as well as glassy and ceramic oxides as solid electrolytes. In each case, HTP screening of substituents (up to 59 dopants tested simultaneously) yields materials with significantly improved properties and also greatly advances our fundamental understanding of the complex role of composition in these systems.
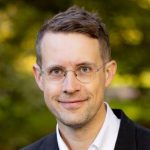
Erik Berg
Professor of Chemistry
Uppsala University
Sweden
Erik J. Berg is since 2021 Professor of Chemistry at Uppsala University-Sweden. He holds a MSc Physics degree from TU Darmstadt-Germany, an Engineering Physics degree from the Royal Institute of Technology-Sweden in 2007 and earned in 2012 a Ph.D. at Uppsala University. He joined the Paul Scherrer Institute, Switzerland as post-doctoral fellow in 2012 where he later was also promoted to Group Leader in 2016, awarded tenure in 2017, before returning to Uppsala University in 2018. He is currently a Knut and Alice Wallenberg Academy Fellow and SSF Future Research Leader. Erik’s research focuses since >10 years on fundamental mechanistic understanding of the chemistry governing the performance of rechargeable batteries. His research team primarily develops and applies operando characterization techniques to study battery dis-/charge processes in real-time, often in close collaboration with industrial funding partners.
Progress on the development of novel battery chemistries is hindered by the lack of understanding of the fundamental mechanisms behind solid electrolyte interphase (SEI) formation and operation. The heterogeneity, nanoscale dimensions, and transient nature of interphasial compounds introduce complexity which for a long time severely impeded the analysis and the consistent description of the SEI. Recent advances in operando interface characterization techniques do however open up for deeper insights into the underlying processes. Herein, model studies of typical Li-ion battery interphase-forming compounds (organic cyclic carbonates, borates, silanes,..) characterized with our operando experimental platform combining online electrochemical gas analysis, quartz crystal microbalance with dissipation monitoring, and infrared spectroscopy will be presented. Focus will be on how vinylene carbonate and electrolyte additives alike effectively suppress the formation of organic compounds, limit interphase thickness growth and rather fill up the porosity of a more compact SEI.
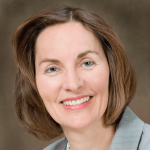
Dr. Esther S. Takeuchi
Professor and the William and Jane Knapp Chair in Energy and the Environment
Stony Brook University
United States
Dr. Esther S. Takeuchi is a SUNY Distinguished Professor and the William and Jane Knapp Chair in Energy and the Environment at Stony Brook University. She holds a joint appointment at Brookhaven National Laboratory as Chief Scientist and Chair of the Interdisciplinary Science Department. Previously, she was employed at Greatbatch, Inc., where her work was instrumental in the development of the lithium/silver vanadium oxide battery, the power source of life-saving implantable cardiac defibrillators. Dr. Takeuchi is a prolific inventor with > 150 patents.
Dr. Takeuchi is a nationally and internationally recognized scientist. She is a member of National Academy of Engineering, the National Inventors Hall of Fame, the American Academy of Arts and Sciences, is a Charter Member of the National Academy of Innovation was awarded the National Medal of Technology and Innovation. She received the E. V Murphree and Astellas Awards from the American Chemical Society and the Electrochemical Society (ECS) Battery Division Technology award. She is a Fellow of the ECS, the American Institute of Medical and Biological Engineering, and the American Association for the Advancement of Science. She has received the European Inventor Award, the Sigma Xi Walston Chubb Innovation Award, an honorary Doctorate in Engineering from Notre Dame University, the ECS Edward G. Acheson Award and was elected to the American Academy of Arts and Sciences. She is the recipient of the 2022 National Academy of Sciences Chemical Sciences Award. She recently received the Yeager Award from the IBA - International Battery Materials Association and the DOE Energy Achievement Award from the Secretary of Energy.
A recent strategy for active material design is the exploration of high entropy oxides (HEOs). HEOs consist of multiple cations within a single oxygen lattice framework. HEOs are an attractive and expansive class of materials due to the exhaustive combination of cation combinations and synthetic approaches that are possible to tailor material properties. The formation of a single phase is based on the concept of entropy stabilization where the random arrangement of multiple components increases the configurational entropy (∆Sconfig) to stabilize the single-phase structure. The configurational entropy of HEOs can be determined by the following
where R is the universal gas constant, and xi and xj represent the mole fraction of the cation and anion components. Materials have been classified as high, medium, or low entropy where high entropy materials have ∆Sconfig ≥ 1.5R, low entropy materials have ∆Sconfig < 1R and materials with 1.5R > ∆Sconfig ≥ 1R are classified as medium entropy. We have synthesized HEO materials using several approaches including targeting several structural forms and compositions. This presentation will examine the resultant electrochemistry as well as the evolution of a material as a function of the redox activity.
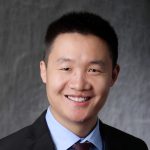
Fudong Han
Professor
Rensselaer Polytechnic Institute
USA
Fudong Han currently holds the Priti and Mukesh Chatter ’82 Career Development Chair Assistant Professorship at Rensselaer Polytechnic Institute, Troy, New York. His research focuses on developing advanced materials for electrochemical energy storage, particularly solid-state batteries. He received his PhD in Chemical Engineering from University of Maryland College Park in 2018. Han is also a recipient of the National Science Foundation CAREER award, the Electrochemical Society Electrodeposition Division Early Career Investigator Award, the Materials Research Society Graduate Student Gold Award, and the Electrochemical Society Battery Division Student Research Award. He has been considered one of the “highly cited researchers” by Clarivate since 2022.
Solid-state batteries (SSBs) are considered one of the most promising technologies for safe and high-energy storage systems. However, key materials issues remain unsolved and serious barriers will need to be overcome for the full-scale commercialization of SSBs. In this presentation, I will introduce our recent work on understanding the key challenges in solid electrolyte materials. We will first discuss the (electro)chemical stability of typical solid electrolytes for enabling next generation electrodes, from iron fluoride cathode, silicon anode, to Li metal anode. We will also present our recent work on understanding the electronic transport in solid electrolytes and its implications for solid-state-battery development.
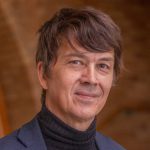
Gerbrand Ceder
Daniel M. Tellep Distinguished Professor of Materials Science
University of California Berkeley
USA
Gerbrand Ceder is the Samsung Distinguished Professor of Engineering at UC Berkeley and a Senior Faculty Scientist at LBNL where he combines theory, computation, machine learning, and experiments to develop novel materials for energy storage. He has published over 500 papers with over 120,000 citations and a Hirsch index of 170. He holds more than 50 US and foreign patents. He is a member of the National Academy of Engineering of the US, the Royal Flemish Academy of Belgium for Science and The Art, and the American Academy for the Arts and Sciences. He is a Fellow MRS, APS, TMS, and ECS, and has received awards from the Electrochemical Society, the Materials Research Society, the Metals Minerals and Materials Society, and the International Battery Association. He is a scientific advisor to multiple companies in the energy storage and materials design space. He leads the Department of Energy program on Earth-Abundant cathode materials (DRX+) and was active in formulating the US Materials Genome Initiative.
DRX materials are of interest as cathode materials as they can be made solely from earth abundant metals such as Mn and Ti and have been shown to display specific energies between 650 to 850Wh/kg at a crystal density near 4g/cc. This gives them higher specific energy and energy density then LiFePO4 and almost at parity with NMC materials. Since high-Mn DRX cathodes lose little or no oxygen when highly charged, they are also likely to provide high safety, which may lead to further benefits at the pack level. Rate capability, which early on posed a problem for DRX cathodes, has been improved through multiple strategies, and 200mA/g and higher rates can now be achieved without substantial capacity loss.
Significant improvements in cycle life have been obtained by creating single crystal DRX with large particle size (multiple microns). The lower surface area of these cathode materials, coupled with modification of the active carbon have enabled operation at high voltage with low capacity fade. I will discuss the activation of these large-crystal materials from DRX to d and discuss in detail how they this transformation improves the voltage profile and rate capability. I will also discuss the remaining challenges to make these materials into commercially viable cathode materials that can provide an earth-abundant and low cost solution for Li-ion technology.
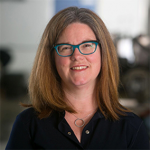
Gillian Goward
Professor
McMaster University
Canada
Gillian R. Goward is a Professor in the Department of Chemistry and Chemical Biology at McMaster University in Canada. Her research team focuses on characterizing the structure and exchange dynamics in materials for energy storage devices including Lithium-, Sodium- and Zinc- Ion Batteries. Characterization strategies implemented by the group include solid-state nuclear magnetic resonance spectroscopy, diffusometry and imaging methods. Our collaborative projects focus on the use of operando detection and quantification of lithium metal plating in LIBs using electrochemical, magnetic resonance imaging, and spectroscopic methods.
Li-ion batteries (LIBs) have become ubiquitous in society; however, improved capacity of LIBs requires increased energy density, achievable through new choices of electrode materials. Beyond mobile devices, a Net-Zero future requires appropriate energy storage for the grid, for which LIBs are impractical. Alternative chemistries are composed of abundant materials and utilize aqueous electrolytes, which are environmentally friendly, sustainable, and cost-effective. Magnetic resonance spectroscopy and imaging techniques are powerful tools for probing dynamic processes in lithium-ion batteries.[1] We have recently reported the application of a parallel-plate resonator to the real-time 7Li operando NMR monitoring of Li metal deposition on a graphite anode during repeated charging and discharging of a single layer prismatic cell.[2] The method allows the quantification of the lithiation of the anode material as well as the early detection of plated metallic lithium throughout the duration of cell charging. Moreover, the assessment of residual plated lithium as compared with plated lithium that can be absorbed during constant voltage holds provides valuable new information regarding the performance of charging protocols. The derivative operando (dOp) method[3] provides excellent visual information regarding the changing speciation in the cell. We have developed a tool which enables efficient deconvolution and quantification of series of NMR spectra. Optimized 1H and 7Li PPRs are utilized to enable high sensitivity in situ and operando NMR experiments with fine temporal resolution, allowing identification of transient species such as Mn2+(aq) dissolution, and accumulation of defect sites.
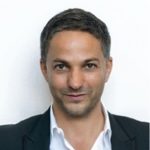
Gleb Yushin
Professor
School of Materials and Engineering at Georgia Institute of Technology
USA
Gleb Yushin is a Regent’s Entrepreneur Professor and Mifflin Hood Chair in the School of Materials Science and Engineering at Georgia Institute of Technology, an Editor-in-Chief for Materials Today (a flagship journal for a family of nearly 200 journals) and Co-Founder & CTO of Sila Nanotechnologies (https://www.silanano.com) (400+ people, multi-billion dollar American manufacturer of advanced materials for Li-ion batteries, including nanocomposite silicon). Gleb has co-authored over 180 peer-reviewed publications, and over 210 patents and patent applications. For his contributions to the development and commercialization of energy storage materials Gleb has received numerous awards and was elected to be a Fellow of multiple organizations: the International Society of Electrochemistry (ISE), the Materials Research Society (MRS), the Electrochemical Society (ECS), the EU Academy of Sciences, and the National Academy of Inventors (NAI). Gleb was also recognized as one of the world’s most influential scientific minds. Gleb holds BS and MS degrees in Physics from Polytechnic Institute and a PhD in Materials Science from North Carolina State University (NCSU).
As conventional Li-ion battery electrode materials approach their theoretical limits, substantial gains in battery energy density only come as a trade-off in safety or performance. Supply chain limitations additionally constrain the use of many conventional materials. This talk will discuss how novel, industry-relevant active and inactive materials may significantly improve performance, reduce the cost of Li-ion batteries, overcome existing and expected supply chain challenges and pave the way for renewable energy technologies. For example, Sila's innovative drop-in-replacement silicon nanocomposite anode powder offers 5-6 times higher gravimetric capacity than graphite, permits both fast charging and over 20% more energy density today over state-of-the-art lithium-ion, and enables radical product innovation - all without compromising performance. This material has been shipping in millions of devices for three years and is scaling up for massive electric vehicle (EV) use by mid-decade. With Sila’s industrialized and scaled scientific innovation, wearables, portable electronics, and EV manufacturers can create breakthrough products today that will minimize our environmental impact tomorrow. This talk will also provide multiple examples of lightweight cathodes, flexible ceramic separators, and other technologies that may become instrumental for the transition to a clean and energy-sustainable economy.
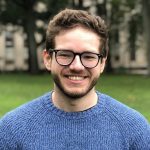
Gustavo M. Hobold
Senior Scientist
SES AI Corp.
USA
Gustavo M. Hobold is a Senior Scientist at SES AI, working on electrolyte discovery for next-generation rechargeable lithium metal batteries. His research focuses on identifying quantitative chemical and functional descriptors of the solid electrolyte interphase (SEI) that can be leveraged for liquid electrolyte design. His research has resulted in the development of a series of experimental high-throughput techniques to precisely characterize and quantify the composition and transport properties of the Li SEI in high Coulombic efficiency electrolytes.
Prior to SES AI, Gustavo obtained a Ph.D. in Mechanical Engineering from the Massachusetts Institute of Technology (MIT), for which he received the 2023 Battery Division Student Award by The Electrochemical Society. He received his Engineering and M.Eng. degrees from Universidade Federal de Santa Catarina, for which he received the ABCM-Embraer Awards for Best Undergraduate Thesis and Master’s Thesis in Mechanical Sciences in Brazil.
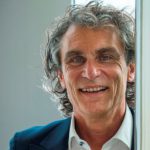
Hubert Gasteiger
Professor
Technical University of Munich
Germany
Hubert Gasteiger received his Ph.D. in Chemical Engineering from UC Berkeley (1993) and was postdoctoral fellow at the Lawrence Berkeley National Laboratory (1994–1995), working with Phil Ross, and Nenad Marković, as well as at Ulm University (1996–1998). Subsequently, he led the development of catalysts and membrane electrode assemblies in the fuel cell program of GM/Opel in the US (1999-2007). After a one year Visiting Professorship at MIT with the group of Yang Shao-Horn, he became Full Professor in the Chemistry Department of the Technical University of Munich (2010). There he founded the Chair of Technical Electrochemistry (https://www.ch.nat.tum.de/tec/home/), focusing on the development of materials, electrodes, and diagnostics for fuel cells, electrolyzers, and batteries. He was editor of Wiley’s Handbook of Fuel Cells, holds 23 patents, and published 282 refereed articles (~68,000 citations, https://scholar.google.com/citations?user=a8P-nVwAAAAJ&hl=de). He is Fellow of the International Society of Electrochemistry (ISE) and of the Electrochemical Society (ECS); he recently received the Vittorio de Nora (2020) and the Battery Division Research Award (2020) from the ECS.
Lithium-ion batteries using Ni-rich layered transition metal oxides (NCMs, NCAs) are widely used CAMs for automotive cells. However, with increasing Ni-content, their stability is compromised at elevated temperatures and high SOC, due to chemical electrolyte oxidation by lattice oxygen release of the CAM and/or by electrochemical electrolyte oxidation at high potentials [1]. Amongst the products of these reactions are protic species (H2O, HF, and H+ [1,2]), which can lead to LiPF6 salt decomposition in the electrolyte [3], active lithium loss via cross-over reactions at the anode, and transition metal dissolution [4,5]. Furthermore, lattice oxygen release leads to the formation of highly resistive oxygen-depleted surface layers on the CAM particles [6]. For these reasons, significant aging of lithium-ion batteries with Ni-rich NCMs/NCAs is not only observed upon extended charge/discharge cycling but also when storing the batteries at high SOC and elevated temperatures.
In this presentation we will discuss the possible reaction mechanisms that lead to these phenomena, making use of conventional battery testing, chemical analysis of the electrolyte, on-line electrochemical mass spectrometry (OEMS), and operando hard X‑ray absorption spectroscopy (XAS).
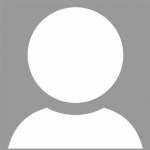
Jacob Haag
Head Of Na And Eu Cam Development
BASF
Canada
Dr. Jacob Haag is BASF’s Head of Cathode Active Materials Development for North America and Europe. He joined BASF in 2013 and has spent the past decade designing, synthesizing and scaling up cathode active materials. Before joining BASF, Dr. Haag earned his PhD from Northwestern University where he studied solid state chemistry and solid oxide fuel cells.
BASF is a leading global supplier of advanced cathode active materials (CAM) for the lithium-ion batteries market, providing high performance CAM to the world’s largest cell producers and for leading platforms of OEMs. In addition, we offer base metals sourcing and management as well as closed-loop battery recycling solutions. By leveraging our industry-leading R&D platforms and passion for innovation, BASF’s Battery Materials and Recycling unit develops unique, proprietary solutions that drive customer success.
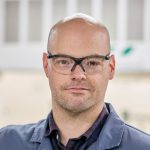
Jean-Christophe Daigle
Lead Researcher
Hydro-Québec, Canada
Canada
Jean-Christophe Daigle completed a Ph. D. in polymer chemistry in 2013 under the supervision of Jérôme P. Claverie at University of Québec at Montréal. His thesis was on development of new polymers based on functional polyethylene. In 2013, he did a trainee in the University of New South Wales in Australia on emulsion polymerization of ethylene under high pressure of carbon dioxide under the guidance of Per Zetterlund. In 2014, he joined to Hydro-Québec for performing a postdoctoral fellow under the direction of Karim Zaghib in IREQ on the development of new polymers applied in batteries. He was a project leader in Esstalion Technologies, a joint venture between Sony Corp. (Murata) and Hydro-Québec, on the development of organic materials for Olivine-based batteries (LFP and LMFP) for energy storage (2014-2018). Currently, J.-C. Daigle is the head of polymers and organic synthesis research group of Hydro-Québec. Moreover, he is the lead researcher of the polymer solid-state battery development program. His main research area is the development of new organic materials, for their application in batteries. These new materials include functional polymers, organic ionic plastic crystals and organic additives. Over the years, he is cumulating more than 50 publications including scientific articles and patents.
Ki Seok Koh,a Annie-Pier Larouche,a Frédéric Roussel,a Francis Barray,a David Lepagea
Chisu Kim,a Armand Soldera,b and Jean-Christophe Daigle*a
a Centre d’excellence en électrification des transports et stockage d’énergie, Hydro-Québec,
1806, Lionel-Boulet Blvd. Varennes, Québec J3X 1S1, Canada
b Laboratory of Physical-Chemistry of Matter (LPCM), Department of Chemistry, Université
de Sherbrooke, 2500 Boul. de l’Université, Sherbrooke, Québec J1K 2R1, Canada
In the race for the electrification of transportation, many companies are competing to develop
more efficient solid-state batteries for commercial applications. Since the landmark discovery
of using polyethylene oxide (PEO) as a lithium-ion carrier for lithium metal batteries by
Armand in 1978, solid-state batteries based on polymer electrolytes have been deeply
scrutinized;1 Viable commercial applications have been provided by Blue Solutions since
2011.2 Despite their many advantages, such as the ability to use lithium metal as a negative
electrode as well as reduced cell weight and enhanced overall safety, the use of polymer
electrolytes remains limited. Additionally, the low overall ionic conductivity and transport
number (t+), poor high-voltage stability, and elevated operating temperature remain obstacles
for their wide implementation in electric vehicles (EVs).
In this presentation, we outline our efforts to develop a high-energy and long-life cycle NMC
– Polymer Electrolyte - Li metal pouch cell. Major challenges are addressed in this pursuit,
including stability of OIPC in positive electrode with NMC, scale-up of the electrode and
performances of the broad range of temperatures.
Major achievement in Hydro-Québec’s development of a new generation high-energy lithium
metal battery was the development of new Organic Ionic Plastic Crystal (OIPC), which was
implemented as catholyte in the electrode formulation. Our new formulation allows the
continuous production of cathode electrode using conventional coating machines used in Liion
battery industry. OIPC is stable with NMC as cathode active material and the formulation
can be easily processed as a biface electrode. Furthermore, we investigated the mechanism of
diffusion involving OIPC, LiFSI and PVDF. The unique mechanism of diffusion opening the
path for developing a new generation of the positive electrode. Based on the unique properties
of the new OIPC family, we achieved high C-rate with a good capacity retention more than
500 cycles at C/6 – 1 C.3
References
1. Mauger, A.; Julien, C. M.; Goodenough, J. B.; Zaghib, K., Tribute to Michel Armand:
from Rocking Chair – Li-ion to Solid-State Lithium Batteries. Journal of The Electrochemical
Society 2020, 167 (7), 070507.
2. Xu, L.; Lu, Y.; Zhao, C.-Z.; Yuan, H.; Zhu, G.-L.; Hou, L.-P.; Zhang, Q.; Huang, J.-Q.,
Toward the Scale-Up of Solid-State Lithium Metal Batteries: The Gaps between Lab-Level
Cells and Practical Large-Format Batteries. Advanced Energy Materials 2021, 11 (4),
2002360.
3. Daigle, J.-C.; Barray, F.; Guerfi, A.; Fleutot, B.; Garitte, E.; Krachkovskiy, S.; Koh, K. S.
Ionic plastic crystals, compositions comprising same, methods for manufacturing same and
uses thereof. WO2022165598, 2022.
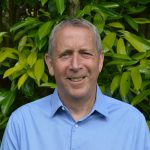
Jerry Barker
CEO
Redoxion Limited
United Kingdom
Jerry Barker received a PhD in solid state electrochemistry from the University of Exeter, UK. In his early career Jerry worked at British Petroleum (BP) and spent time at the University of California, Santa Barbara, (UCSB) collaborating with the Nobel prize winners Alan Heeger and Hideki Shirakawa on alkali-ion doped conducting polymers. As chief electrochemist at BPSolar he developed a process for the large-scale electrodeposition of II-VI semiconductors for photovoltaics applications.
Jerry is currently co-founder and Chief Scientist at Faradion Limited, a UK-based start-up specializing in Na-ion battery technology. Previously Jerry was Chief Scientist and Research Director at Valence Technology Inc.
Jerry has published extensively (h-index = 61, total citations >12,000) and is the named inventor on more than 115 issued US patents. These patents cover numerous alkali ion active materials as well as the Carbothermal Reduction (CTR) volume manufacturing method. The inventions have culminated in five commercially successful battery ventures and CTR is widely-regarded as the benchmark process for the large-scale synthesis of LiFePO4.
In 2012, Jerry was awarded the IBA Technology Award for his contributions to Li-ion battery materials. and received the ECS Europe Section Alessandro Volta Medal in October 2022 recognizing excellence in electrochemistry. He has appeared as a patent litigation expert witness in Europe and in North America. Jerry currently acts as an Expert Panel member for the UK’s Faraday Institution and sits on the advisory board for Australia’s storEnergy initiative. In 2019 Jerry was appointed Honorary Professor within the School of Chemistry at the University of St. Andrews, UK. To target the low-cost and sustainable Li-CAM supply chain, Jerry founded the start-up company, Redoxion Limited in 2022.
Redoxion Limited, The Bagel Factory, 22 White Post Lane, London, E9 5SZ, United Kingdom
Lithium iron phosphate, LiFePO4 has gained wide acceptance as the cathode material of choice for safe, large format Li-ion battery applications. The market for LiFePO4 cathode powder is predicted to reach over $20 Bn by 2030 and there is a general trend towards this cell chemistry among global automotive companies [1]. Since LiFePO4 possesses a low intrinsic conductivity, carbon-coating methods or suitable preparative approaches must be employed to produce a composite product incorporating a conductive component [2]. The Carbothermal Reduction method for the large-scale synthesis of LiFePO4 has been adopted widely by the Li-ion industry over past 20 years [3,4].
In this study we describe new and sustainable solid-state synthesis methods for the volume manufacture of phase pure and highly conductive LiFePO4. These approaches utilize low-cost and earth-abundant Fe precursors and careful consideration has been given to ensuring a secure and reliable supply chain. These novel preparative methods are designed to use industry-standard equipment and production processes, while cost analysis confirms they will be cost-competitive with any currently employed manufacturing method.
In this presentation we will describe the physical and electrochemical performance characteristics of the synthesized LiFePO4 materials. Typically, the cathode material delivers close to theoretical performance and demonstrates extremely low-capacity fade on cycling. In addition, the active material operates with low polarization and with excellent columbic and energy (round-trip) efficiency.
Using an extension of this work, similar preparative approaches have been adopted to synthesize the second-generation material, lithium iron manganese phosphate, LiFe1-xMnxPO4, with particular emphasis being paid to delivering cathodes with Fe:Mn ratios optimized for commercial applications.
References:
[1]. For example, International Energy Agency (IEA) Report, Global Supply Chains for EV Batteries. 2023.
[2]. J. Barker, M.Y. Saidi and J.L. Swoyer, Electrochem. Solid-State Lett., 6, A53, 2003.
[3]. J. Barker, M.Y. Saidi and J.L. Swoyer, J. Electrochem.Soc. 150 (6), A684, 2003.
[4]. J. Barker, M.Y. Saidi and J.L. Swoyer, For example, US Patent 6,528.033 (Issued 2003), US Patent 6,702,961 (Issued 2004) and others. Assignee: Valence Technology Inc.
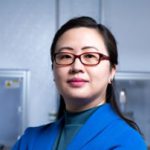
Jie Xiao
Battelle Fellow
Battery Materials & System Group at Pacific Northwest National Laboratory
USA
Dr. Jie Xiao is currently a Battelle Fellow and leads Battery Materials & System Group at Pacific Northwest National Laboratory (PNNL). She is also a PNNL-University of Washington distinguished faculty fellow. Dr. Xiao’s research spans from fundamental research, battery materials scaleup and manufacturing to cell fabrication and engineering for vehicle electrification and grid energy storage. She has published more than 100 peer-reviewed journal papers and been named top 1% Clarivate Analytics Highly Cited Researcher since 2017. She holds seventeen patents in the area of energy storage area and seven of them have been licensed to industry. She is the recipient of a few awards such as US Department of Energy’s E. O. Lawrence Award, Battelle Distinguished Inventor and Fellow of The Electrochemical Society etc.
Identifying and addressing material challenges at industry-relevant scales and validation of new battery chemistries under realistic conditions critically determine the timeliness and success of materials development, manufacturing, and technology translation from academic research to industry applications in the US. There remains to be a large gap between academic research, materials scale-up/manufacturing, and device level performance optimization.
This talk will review the challenges, opportunities, and approaches for accelerating R&D and manufacturing processes of next generation materials and battery technologies. I will highlight the importance of interdisciplinary research in electrochemical energy storage and emphasize the necessity to identify and address scientific challenges at relevant scales/conditions. Two specific examples will be discussed: (1) an integrated electrochemistry and engineering approach to utilize lithium metal anode and enable high-energy rechargeable lithium metal battery, (2) the study of single crystal Ni-rich cathode for Li-ion and Li metal batteries. Scaling up single crystal cathode will be used as an example to shed some light on the importance of integrated science and engineering methodology for battery materials development and manufacturing.
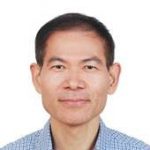
John Lin
President
E-Car Solution Center (CATL)
China
Dr. Yong-Shou (John) Lin is the president of E-Car Solution Center at CATL, China. He has more than twenty years of experiences in the Li-ion battery industry, specializing on Li-ion battery material & electrolyte development, cell design, and battery safety technology. He received his PhD from the University of Valladolid, Spain in 1995 before being awarded a JSPS post-doctoral fellowship to research at Waseda University, Japan. Before joining CATL, he was the general manager of R&D at E-One Moli Energy Limited (Canada). He has published over 30 papers, 2 book chapters, and more than 30 patents.
Power and transportation are two critical CO2 emission sectors. Li-ion battery is becoming as an important technology to be applied in green energy and transportation electrification for achieving carbon neutralization. Recent battery technology innovations will be discussed.
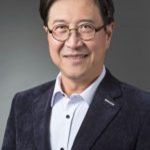
John Zhang
CTO/CSO
Polypore
USA
John is recognized as the leading authority on lithium-ion battery safety and separators. He is a leader in establishing lithium-ion battery industry standards (P1625, P1725, and CTIA) through the Institute of Electrical and Electronics Engineers (IEEE). He has chaired and/or organized more than 60 international conferences and delivered more than 60 invited keynote or plenary speeches at various international conferences. John has published more than 192 patents, >150 papers, and 4 book chapters, including the first ceramic coating separator patent (US 6, 432, 586) and some of the most cited papers (Chem. Reviews, 2004 and Li-ion safety papers 2006-now). Dr. Zhang received Ph.D. in Material Chemistry from the University of California, Santa Barbara.
While cathodes, anodes, and electrolytes have basked in the limelight, one of the 4 KEY components of the lithium batteries, SEPARATOR has long been cloaked in secrecy, although it has been silently guard the heart of lithium batteries, a key player in the pursuit of safety, high energy density and long cycle life.
Earlier stage of Li batteries, especially high energy density batteries showed frequent fire and explosion (1000 ppm to 10 ppm) during normal uses, normal abuses and transportations, which seriously affected human safety and limited the Lithium battery progress.
Initial study of Li battery fire and explosion showed the necessity of Ceramic Coated Separator (CCS) to greatly improve the safety of Lithium batteries. The CCS was invented during 1998 and patented at 2000 (Patent 586).
Immediately after the adoption of ceramic coated separators during 2003-2005, the fire/explosion of Li batteries was reduced to 1 ppm - < 0.01 ppm, CCS have redefined Li battery safety. By significant improvement of Li battery safety, CCS also enabled high energy density Li batteries due to (more active material and higher energy) their much more frequent fire and explosion. With CCS interactions with H2O, HF and many harmful cycling by-products and its interactions with dendrite the cycle life of Li batteries also have been greatly improved.
Now, CCS enabled <1 ppb on lithium battery fire and explosion. In turn, 100% of Cell phone and notebook PC has to use CCS, 97% NMC type high voltage cathode Li batteries has to use CCS, another 60% LFP type of EDV batteries is in the transition from normal separator to CCS.
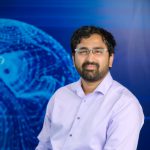
Dhevathi Rajan Rajagopalan Kannan, Ph.D
Research Scientist
UL Research Institute
USA
Dr. Dhevathi Rajan Rajagopalan Kannan is a Research Scientist in the Electrochemical Safety Research Institute (ESRI) at UL Research Institutes (ULRI). Dr. Kannan has more than six years of experience in battery research, battery cell testing and validation, and modeling and simulation.
Before joining ESRI, Dr. Kannan worked with QuantumScape Battery, Inc., in San Jose, California, as a Senior Battery Test Engineer. He supported various research and development projects on solid-state batteries, which included designing experiments, cell teardown efforts for electrode analysis, safety tests and benchmarking, data analysis to understand the solid-state battery electrical behavior and performance, and other related research activities. Prior to that, Dr. Kannan worked with Romeo Power, Inc., (acquired by Nikola Motor Company) as Manager of Battery Cell Testing and as a Battery Test Engineer.
Dr. Kannan earned his Ph.D in Electrical Engineering and a Master of Science degree in Electrical Engineering from the FAMU-FSU College of Engineering, Florida A&M University, Tallahassee, Florida (2020 and 2018). He completed his Bachelor of Engineering degree in Electrical and Electronics Engineering from Anna University, Tamil Nadu, India in 2015 and won the Academic Excellence Award in 2011-2012.
The Electrochemical Safety Research Institute (ESRI) has been carrying out safety and performance characterization tests on commercial sodium-ion cells. Cells from two manufacturers were procured. The operating voltage of cells from manufacturer A was 1.5 V to 3.95 V and from manufacturer B was 1.5 V to 4.1 V. Single cells were subjected to overcharge, overdischarge, external short circuit and heating tests to characterize their performance under these off-nominal conditions. Cells were also connected in series to form 12 V and 48 V modules to study the off-nominal behavior in multi-cell configurations. Electrodes in the commercial cells were analyzed for composition and it was found that apart from sodium, the cathodes contained metal additives such as Li, Mn, Mg, Al, Ni, Fe and V for manufacturer A and Li, Mg, Mn, Fe, V, Ni and Zr for manufacturer B. The cells from manufacturer A were tolerant to overcharge, overdischarge and external short tests. The cells from manufacturer B had one cell that vented electrolyte under the overcharge test but had similar behavior to manufacturer A for the overdischarge and external short tests. Under the heating tests, cells from manufacturer A experienced thermal runaway with extrusion of cell contents at about 149 °C and while those for manufacturer B experienced thermal runaway at about 139 °C with maximum temperatures recorded being 305 and 450 °C respectively (Figure 1). The results of the performance of the single cells under two different rates as well as the results of the safety tests at the module level will also be presented.
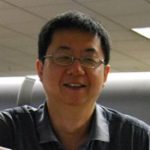
Kang Xu
Chief Scientist
Solid Energy Systems
USA
Kang Xu is an MRS Fellow, ECS Fellow, ARL Fellow (emeritus), and former team leader at Battery Science Branch of DEVCOMM Army Research Laboratory in Adelphi, Maryland. He received Ph. D. in Chemistry under the tutelage of Prof. Austen Angell at Arizona State University, and has been conducting electrolytes and interphasial chemistry research for the past 30 years. He has published 300+ papers, wrote/edited 5 books/chapters, and obtained 20+ US Patents, with total citation of 62,000+ and an h-index of 122. He is a Clarivate’s highly-cited author, and one of the top 2% most influential researchers in the Stanford Database.
Besides the numerous publications, he is best known in the field for the two comprehensive reviews published at Chemical Reviews in 2004 and 2014, and a book entitled “Electrolytes, Interfaces and Interphases” published by RSC Press in April 2023. His work has received many recognitions and awards within DoD and in the field, including multiple Depart of the Army R&D Awards, the 2015 UMD Invention of the Year, 2017 International Battery Association Technology Award, and 2018 ECS Battery Research Award. Upon his retirement from federal service 2023, he received an Army Civilian Service Medal.
In 2023 Summer, he joined SES AI Corp and serves as its Chief Scientist.
Electrolyte plays the central role in all electrochemical devices, and it is the only component in such devices that physically interacts with every other component, be it electrochemically active (anode and cathode) or inert component (separator, substrate, binder, conducting additive, packaging etc.). Whenever there is interaction, an interface arises, and whenever such interaction occurs at electrochemical potential far away from the thermodynamic equilibria, an interphase becomes evitable.
This talk will summarize my recent work in designing better interphases for advanced battery chemistries, be it Li-ion, Li-metal or other battery chemistries.
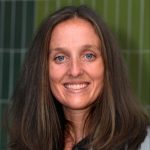
Kelsey Hatzell
Assistant Professor
Princeton University
USA
Dr. Hatzell is an assistant professor at Princeton university in the department of Mechanical and Aerospace Engineering and the Andlinger Center for Energy and the Environment, At Princeton University, Dr. Hatzell leads the Materials for Energy and Climate Lab (HatzellLab.Princeton.edu). The group primarily works on energy storage, energy conversion, and separations applications and is particularly interested at using non-equilibrium x-ray techniques to probe materials during in situ and operando operating conditions. .Dr. Hatzell earned her Ph.D. in Material Science and Engineering at Drexel University, a M.S. in Mechanical Engineering from Pennsylvania State University, and a B.S./B.A. in Engineering and Economics from Swarthmore College. Hatzell is the recipient of several awards including the ORAU Powe Junior Faculty Award (2017), NSF CAREER Award (2019), ECS Toyota Young Investigator Award (2019), finalist for the BASF/Volkswagen Science in Electrochemistry Award (2019), the Nelson “Buck” Robinson award from MRS (2019), Sloan Fellowship in Chemistry (2020), and POLiS Award of Excellence for Female Researchers (2021), NASA Early Career Award (2022), the ONR Young investigator award (2023), and Alfred Rheinstein Award (2023).
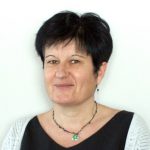
Dr. Laurence Croguennec (ICMCB-CNRS)
Senior Researcher/Deputy director
Institut de Chimie de la Matière Condensée
France
Laurence Croguennec is a CNRS Research Director at the Institut de Chimie de la Matière Condensée (ICMCB-CNRS, France) at the Bordeaux University. She graduated (PhD) in 1996 from Nantes University at the Institut des Matériaux Jean Rouxel (France) and spent one year as a Post-Doc at the Bonn University (Germany). She became CNRS researcher at ICMCB in 1997, led the research group “Energy: Materials and Batteries” between 2004 and 2021 and is Deputy Director of ICMCB since 2022. She is also actively involved in the French Network on the Electrochemical Energy Storage (RS2E), in the ALISTORE European Research Institute devoted to battery research and in the France 2030 acceleration program with the PEPR batteries.
Laurence Croguennec has been working for more than 25 years now on the crystal chemistry of electrode materials developed for Metal-ion batteries, and more recently all-solid-state batteries, and on the characterization of mechanisms involved upon their cycling, especially for layered and spinel oxides and polyanionic-type positive electrode materials. She develops also research in collaboration with European neutrons and synchrotron large scale facilities for in situ and operando characterization of materials during the operation of the batteries. She is the co-author of more than 160 publications, 5 book chapters and 5 patents in this field; she delivered more than 80 invited talks, and organized 18 international and 7 national meetings or symposiums.
During this talk, I will highlight about new attractive phases and mechanisms identified in the systems Na3V2(PO4)3 and KVPO4F1-yOy, as well as about the key role of defects and extent of ordering on the electrochemical performance of high-voltage spinel:
- A new class of NASICON phases NaxV2(PO4)3 has been obtained. On the contrary to conventional Na3V2(PO4)3, a slopping voltage profile is obtained at a higher voltage of 3.6 V vs. Na+/Na, with an extremely small polarization and great capacity retention.
- New potassium and vanadium oxyfluoride phosphates of the KTiOPO4 structural type and with a chemical composition KVPO4F1-yOy (0 ≤ y ≤ 1) have been synthetized. In particular, the compound KVPO4F5O0.5 is promising.
- The unique ability to tune the primary particle morphology, spinel composition and secondary phase generation in spinel LiNi1/2-xMn3/2+xO4 will be demonstrated. 4D-STEM was used to dissect the structure at the nanometric spatial resolution, and heterogeneity in the transition metal arrangement of the globally ordered (P4332) LiNi1/2Mn3/2O4 was shown beneficial for electrochemical performance.
I will demonstrate that only the in-depth control of the relationship synthesis/composition/atomic and electronic structure allows to tune the properties in the battery.
- Keywords
Metal-ion batteries, positive electrode materials, polyanionic materials, high voltage spinel oxides, structural and redox processes
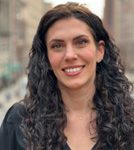
Lauren Marbella
Principal Investigator
Columbia University
New York
Lauren Marbella is an Associate Professor in the Department of Chemical Engineering at Columbia University. Her research group focuses on understanding the relationship between electrochemical performance and interfacial chemistry in devices for energy storage and conversion. Her research relies heavily on the use of nuclear magnetic resonance imaging (MRI) and spectroscopy to evaluate changes in material properties in real time to elucidate the chemical mechanisms underpinning degradation in Li and beyond Li-ion battery systems. Marbella’s research has received numerous awards including the ACS Materials Au Rising Stars in Materials Research Award (2022), Cottrell Scholar Award (2022), the National Science Foundation (NSF) Faculty Early Career Development (CAREER) Award (2021), and the Scialog Collaborative Innovation Award for Advanced Energy Storage (Sloan Foundation, 2019).
She received her PhD in chemistry from the University of Pittsburgh in 2016, under the direction of Prof. Jill Millstone. In 2017, she was named a Marie Curie Postdoctoral Fellow at the University of Cambridge in the group of Prof. Clare Grey. There, she was also named the Charles and Katharine Darwin Research Fellow, which recognizes the top junior fellow at Darwin College at the University of Cambridge. She joined the chemical engineering faculty at Columbia University in 2018.
Despite the fact that the solid electrolyte interphase (SEI) on Li metal was described 45 years ago, it is still the only aspect of the battery that has ambiguity in function. As a community, we have struggled to establish structure-property-performance relationships for the SEI because it is a nanoscale composite that contains chemical compounds whose properties deviate from their bulk counterparts. In this talk, I will describe how we have used nuclear magnetic resonance (NMR) spectroscopy to characterize the structure and dynamics of interfacial phenomena in Li-ion and beyond Li-ion batteries and correlate these features with battery performance. In particular, I will focus on the use of NMR to quantify the source of Li inventory loss, the mechanism of transition metal dissolution, structural evolution at the electrode/electrolyte interface, and the function of the SEI. Insight from these methods allow us to determine the precise mechanisms of failure that arise inside of functional devices as well as develop new approaches to mitigate performance decline.
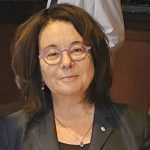
Linda Nazar
Distinguished University Research Professor of Chemistry
University of Waterloo
Canada
Linda Faye Nazar FRS FRSC OC is the Senior Canada Research Chair in Solid State Energy Materials and Distinguished University Research Professor of Chemistry at the University of Waterloo, Canada. She is a Fellow of the Royal Society (London), the Royal Society of Chemistry (UK), an Officer of the Order of Canada, and a Justus Liebig Visiting Professor in Germany.
Professor Nazar’s research focuses on developing new electrochemical energy storage materials, and on advancing next-generation rechargeable battery research through a combination of materials synthesis, advanced characterization tools; electrochemistry and modelling.
She has co-authored ~ 300 publications garnering more than 80,000 citations (H index 128; Google Scholar). She has been a member of the Directorate of the USA Department of Energy’s Joint Center for Energy Storage Research since 2013. Prof. Nazar currently sits on the Advisory Councils for the Andlinger Center for Energy and the Environment at Princeton University, and the Israel National Research Centre for Electrochemical Propulsion. She is the Director of the Waterloo Institute of Technology’s International Scientific Advisory Board.
Professor Nazar has received numerous awards, including the Materials Research Society Medal (2020, co-awarded with Yi Cui); the Bailar Medal (Univ Illinois UC, 2019); the IUPAC Distinguished Woman in Chemistry (2011); and the Moore Distinguished Scholar (California Institute of Technology) (2010). Other recognitions include national awards such as those from the Chemical Society of Canada (the CIC Medal: 2019 and the Steacie Award, 2022) and international accolades including the Electrochemistry Society Battery Division Research award (2009), the International Battery Association award (2011), the August-Wilhelm-von-Hofman Lecture award (German Chemical Society, 2013), and the International Automotive Lithium Battery award (2017).
All-solid state Li-ion and Li-S batteries (ASSBs) have emerged as very attractive alternatives to conventional liquid electrolyte cells for e-mobility, owing to their enhanced safety and higher energy densities. Similarly, low-cost solid state Na-ion batteries may prove an promising alternative for stationary applications.
ASSBs are founded on high performance fast-ion conducting electrolytes, and in the important search for new materials, alkali thiophosphates (sulfides) and (oxy)halides are particularly promising classes of materials owing to their excellent mechanical properties. Sulfide-based solid catholytes are especially promising materials for high-performance solid-state sulfur cells that operate on the reversible conversion of S ↔ Li2S. However, their electrochemical decomposition above 2.5 V vs Li+/Li causes progressive degradation in the operating window of the cell, because the free S2- ions are oxidized to sulfur at a similar potential as Li2S. Oxyhalides show particular advantage owing to their good interface stability with high voltage NMC-type cathodes.
The talk will present an overview of the state-of-the art in the field, followed by a focus on recent findings in our laboratory and the understanding of superionic conductivity in these materials using a combination of structural elucidation via X-ray/powder neutron diffraction, pair distribution function analysis, ion conductivity mechanisms, and ab initio molecular dynamics simulations. We correlate crystal structure with ionic conductivity in a range of our newly developed fast ion Li and Na conductors. These considerations lead to an overarching understanding of the relative importance of interstitial alkali ion migration, defect concentration, and the factors that govern thermodynamic (meta)stability. The talk will highlight their application in solid state batteries and factors that govern the cathode-electrolyte interface.
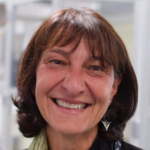
Maria Forsyth
Professor
Deakin University
Australia
Maria Forsyth AM ATSE (Australian Academy of Technology and Engineering), FAA (Fellow Australian Academy of Sciences), is a Deakin Distinguished Professor at Deakin University and an Ikerbasque Visiting Professorial Fellow at University of the Basque Country, Spain. Professor Forsyth has worked at the forefront of energy materials research since her Fulbright Research Fellowship in 1990 and has consistently made breakthrough discoveries, including in polymer electrolytes, ionic liquids and organic plastic crystals. Her research has focused on understanding the phenomenon of charge transport within these materials and also at metal/electrolyte interfaces present in all electrochemical applications., and in particular Li and Na batteries. She has supervised over 100 PhD students and is a co-author of over 880 journal and conference publications, attracting more than 40000 citations.
Since the early reports of Aluminium electroplating from imidazolium chloride ionic liquid electrolytes in the early 1990s, organic cation based liquid salts have been considered as promising materials for metal electrochemistry, and in particular, for applications in high energy density alkali metal batteries including Lithium and Sodium. With the discovery of the bis(trifluoromethanesulfonyl)amide and the smaller bis(fluorosulfonyl)amide analogue (FSI) ionic
liquidliquids, a range of ‘solvents’ based on ammonium, pyrrolidinium and phosphonium chemistries have been explored for these applications with varying success. In recent years, it has been discovered that mixtures of ILs with high concentrations of the alkali metal salt (eg LiFSI or NaFSI) provide unique coordination environmentsenvironments, ion transport mechanisms and interfacial structure that leads to stable, reversible electrochemical cycling of metal anodes. This has paved the way to development of commercially relevant, high performance and safe batteries based on these electrolytes. Many of these
systems also support the use of high voltage, high capacity layered oxide cathode materials which
further increase battery energy density and potential applications where these attributes are important.
In this talk we will explore the impact of chemical composition and the unique properties of IL
electrolytes, taking into account both early learnings and newer developments, that demonstrate IL
based electrolytes are becoming viable for high performance batteries. Despite the historical cost
concerns, through engineering of more sustainable manufacturing methods such as flow chemistry,
scale up of these materials is being actively pursued by chemical companies, thus providing the pathway to more competitive pricing and ultimately commercial reality for IL based batteries
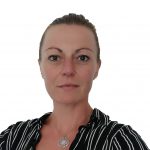
Margaud Lécuyer is Head of Electrochemistry Innovation at Blue Solutions, a subsidiary of the Bolloré Group and global designer and manufacturer of all-solid-state batteries. She joined the company in 2010.
Since 2017, she has been in charge of innovation topics, overseeing the development of new chemistry and materials to be incorporated in future generations of cells. Among other tasks, she designs and monitors R&D projects, actively pursues funding and partnerships, promotes new technologies among OEMs, manages the laboratory and supervises staff. She was previously responsible for production monitoring, acting as interface between the R&D, production, and quality departments, and was tasked with approving battery packs.
Margaud Lécuyer has an engineering degree from ESPCI Paris, specializing in physics, and a PhD in materials chemistry from the Institut des Matériaux Jean Rouxel in Nantes, focusing on lithium-sulfur and lithium-organic batteries.
Blue Solutions is already known as the pioneer company in the field of solid-state batteries, having put on the market Lithium Metal Polymer batteries since 2011. However, previous generations only addressed heavy duty applications, with their high working temperature, around 80°C. Currently, we are preparing next generation by developing a new electrolyte chemistry. This electrolyte is still polymer-based but delivers a sufficiently high ionic conductivity at room-temperature. In addition to that, Blue Solutions latest strategy has been to develop in parallel three different options based on three active materials, allowing to address all the passenger car segments, from basic to premium cars. In this talk we will share the latest results got with this 4th generation under optimization, and our roadmap until final industrial volumes.
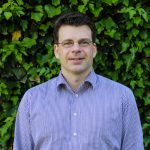
Mark Obrovac
Researcher
Dalhousie University
Canada
Dr. Obrovac has a BSc in Chemical Physics (Simon Fraser University, 1995), a MSc in Physics (Dalhousie University, 1997), a PhD in Physics (Dalhousie University, 2001), and was a Postdoctoral Fellow in Chemistry at Cornell University (2001-2002). In 2002, Dr. Obrovac joined 3M Company, St. Paul Minnesota, as a Research Specialist and Li-ion Battery Anode Materials Project Leader. There he was responsible for new battery materials research, development, scale-up, and manufacture. In 2010, Dr. Obrovac became professor of Chemistry at Dalhousie University with cross appointments to the Departments of Physics and Process Engineering and Applied Science. Dr. Obrovac's research group at Dalhousie University conducts research on sustainable Li-ion and Na-ion battery materials and their synthesis methods.
Dry processes have emerged as important synthesis methods for Li-ion battery materials because of their low cost and sustainability. Dry processes can also make new and unique materials. Recent interest in dry processing has led to new methods that can synthesize engineered particles with morphologies that are not accessible by other means, leading to further advances in Li-ion battery materials development. As examples, we have recently shown that single-crystal NMC cathodes may be made using all-dry methods and we have also shown that by applying dry mechanofusion processing, low surface area LiFePO4 cathode can be made that can achieve >30% higher energy density than conventional materials. This presentation will summarize advances in all-dry processing for cathode and anode materials synthesis, including the utilization of mechanofusion processing to make coated particles, embedded particles, and high energy density cathode particles.
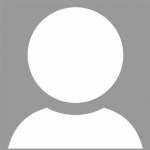
Matthieu Morcrette
Tiamat Energy
France
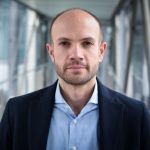
Matteo Bianchini
Professor
University of Bayreuth
United Kingdom
Matteo Bianchini studied physics engineering at Politecnico di Milano and obtained his PhD in Solid State Chemistry in 2015 with Prof. Masquelier, Dr. Croguennec and Dr. Suard in a collaboration between ILL (Grenoble), LRCS (Amiens), and ICMCB (Bordeaux). He was later a postdoctoral researcher for two years in the group of Prof. G. Ceder at the Lawrence Berkeley National Lab, where he worked on the design, synthesis, and characterization of new materials for next generation Na- and K-ion batteries. He was then a postdoctoral researcher at BELLA-KIT (Prof. J. Janek), where he investigated cathode materials for Li-ion batteries, prior to joining BASF as a lab team leader to co-supervise the BELLA lab. In 2021 Matteo obtained the chair of Inorganic Active Materials for Electrochemical Energy Storage at the University of Bayreuth, where he has built his group to continue investigating and developing electrode materials for the next generation of batteries.
Layered oxides NaxMO2 are among the most attractive cathode active material candidates for Na-ion batteries. P2 and O3 are well-known polytypes in the NaxMO2 system. P2 is believed to be preferable over O3 regarding Na+ conductivity, yet P2 materials can only be synthesized with low Na content (x = 0.67-0.7), which is problematic when building full cells. Another issue of P2 is the P2–O2 phase transition taking place at high voltage during electrochemical cycling, which is not reversible and limits capacity retention. The aim of our work is to maximize the Na inventory available for P2 materials, and to improve the cycling stability by doping inactive elements in the pristine materials without excessively compromising discharge capacity. In this presentation, we focus on a family of Li-doped P2 materials with nominal compositions of Na5/6LiyNi5/12-3y/2Mn7/12+y/2O2 (y = 2/18, 3/18, 4/18, 5/18). We report on the characterization of the crystal structures of these materials, as well as the temperature-resolved in situ XRD during their solid-state synthesis.
Electrochemically, this family of materials show an increasing trend of polarized hysteresis in the first cycle, which suggests capacity contribution from oxygen redox. We coupled semi-simultaneous operando XANES and XRD to understand the structural evolution and redox behavior during this process, as well as OEMS to detect the amount and onset of oxygen release. In particular, we observed that a Li content higher than 0.15 per formula unit in the TM site mitigates the P2-O2 phase transition at high voltage and facilitates the activation of the O-redox starting at lower voltages.
The cycling stability and rate performances of these materials were investigated in two and three electrode half cells, also investigating the role of distinct particle morphologies. Finally, we applied the best materials against home-made hard carbons in exploration of their performances in full cells.
Prof. Mauro Pasta is Professor of Applied Electrochemistry in the Department of Materials at the University of Oxford. He currently serves as the Principal Investigator of the SOLBAT (solid-state batteries) project as part of The Faraday Institution. His research interests encompass electrochemistry and materials chemistry, with a primary focus on developing innovative materials for electrochemical energy storage. As a co-founder of three battery startup companies, Natron Energy, Cuberg and Project K, Prof. Pasta is dedicated to translating his research into real-world solutions.
Potassium-ion batteries are emerging as a promising complementary technology to lithium-ion batteries due to their prospective low cost and potential high-rate capability1. In my talk, I will discuss the progress our group has made in characterising the transport and thermodynamic properties of K-ion electrolytes2, the structure-electrochemistry relationship in Prussian Blue analogue cathodes and graphite anodes3,4, and in modelling full cell performance5.
References
- Dhir, S., Wheeler, S., Capone, I. & Pasta, M. Outlook on K-Ion Batteries. Chem 6, 2442–2460 (2020).
- Dhir, S., Jagger, B., Maguire, A. & Pasta, M. Fundamental investigations on the ionic transport and thermodynamic properties of non-aqueous potassium-ion electrolytes. Nat. Commun. 14, 3833 (2023).
- Cattermull, J., Pasta, M. & Goodwin, A. L. Structural complexity in Prussian blue analogues. Materials Horizons vol. 8 3178–3186 (2021).
- Cattermull, J., Roth, N., Cassidy, S., Pasta, M. & Goodwin, A. K-ion slides in Prussian Blue Analogues (2023).
5. Dhir, S. et al. Characterisation and Modelling of Potassium-ion Batteries. Research Square (2024).
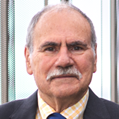
Dr. Michel Armand
Scientific Director
Cic Energigune
France
Prof. Dr. Michel Armand received his Ph.D. in Physics from University Joseph Fourier (France) in 1978. He was Director for Research at the Centre National de la Recherche Scientifique (CNRS, France) since 1989 and Professor at University of Montreal (1995−2004). He has ushered theoretical concepts and new materials leading to practical applications in energy-related electrochemistry. He has been one of the pioneers of the use of intercalation electrodes (1972), and ushered the concept of “rocking chair” batteries using a second intercalation compound at the negative electrode, which will become known as the lithium-ion. He has introduced polymer electrolytes (1978) which are now used in the first commercial solid-state battery in application, new salts based on delocalized anions, of the sulfonimide family like TFSI or FSI (1986), widely used in batteries and the base for ionic liquids; he developed the first organic electrode materials (1996) and the carbon coating for LiFePO4, making it the most popular and safest electrode materials in battery production. He joined CIC Energigune in 2011, and present activities include new solvating polymers for lithium and sodium, lithium sulfur batteries, and several new salts. Michel Armand has been an author or a co-author for > 500 publications while contributing to 16 book chapters, has a H factor of 110 and his work has been quoted > 95000 times. He is the recipient of several distinctions and honours including the silver medal (CNRS), the Faraday Division Award from the Royal Society (UK), the Pergamon and Volta gold medals. Michel Armand is an ECS Fellow.
Centre for Cooperative Research on Alternative Energies (CIC energiGUNE), Basque Research
and Technology Alliance (BRTA), Álava Technology Park, Albert Einstein 48, 01510 Vitoria-
Gasteiz, Spain. [email protected]
Lithium and sodium batteries, the first with an established market and the second with a
foreseen future in grid storage at least, are likely to see a dramatic increase in volumes produced,
in term of tens of million tons. Solid Electrolytes (SEs) are seen as a possible solution for
harnessing the metal (Li°, Na°) negative electrode. Already, polymer electrolytes are
successfully implemented in batteries comprising the sequence Li°| PEO electrolyte| LiFePO4
by the company Blue Solutions. Only a moderate pressure is needed to operate the lithium metal
electrode (≈ 2 bars) due to the ad-hoc mechanical properties and adhesion of the polymer.
Ceramic electrolytes (Argyrodite, garnets…) have conductivities 3 orders of magnitude higher
at room temperature but need enormous pressures (tens of bars) to operate to accommodate the
volume changes inherent to mass transfer at the electrodes.
The race to improve the conductivity of polymers at room temperature is two-pronged: i)
staying with dry polymer electrolytes (solvent-free), in a change of polymer architecture; ii)
resorting to gels, i.e. the plasticization of a polymer matrix with a liquid polar solvent. The first
strategy is based on either comb polymers with improved chain flexibility, but these systems
are still “coupled”, i.e. the ion mobility is varying in the same way as the microscopic viscosity,
above Tg. Interestingly, poly(zwitterions) as a host matrix are newly appearing as decoupled
systems, combining excellent mechanical properties and decent conductivities. Gels, on the
other hand, can reach high conductivities and the polymer is mostly conferring the mechanical
properties, but the problem of SEI/CEI formation and crosstalk between the electrodes is
reminiscent of that seen in liquid electrolytes.
All these strategies will be appraised in detail in this presentation.
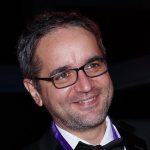
Mircea Dinca
M. Keck Professor of Energy and Professor of Chemistry
Massachusetts Institute of Technology, Department of Chemistry
USA
Mircea Dincă was born in Făgăraş, a small Transylvanian town in central Romania. He obtained his Bachelor of Arts degree in Chemistry from Princeton University in 2003, and did his graduate work at UC Berkeley, where he obtained a PhD in Inorganic Chemistry in 2008. After a two-year stint as a postdoctoral associate working on heterogeneous electrocatalytic water splitting at MIT, he became an Assistant Professor in the Department of Chemistry at MIT in July 2010. Promoted to Associate Professor in 2015 and to Professor of Chemistry in 2020, he currently holds the W. M. Keck Chair as Professor of Energy at MIT. His research interests focus on creating and manipulating microporous and low-dimensional solids with molecular precision for applications in various energy efficiency and environmental challenges. These include, but are not limited to: electrical energy storage and conversion, heterogeneous catalysis, fresh water harvesting, efficient air conditioning, and photophysical processes. He has been named to the world’s most cited Chemists list annually since 2014 and has received a number of awards, most prominently the Alan T. Waterman Award (2016), the ACS Award in Pure Chemistry (2018), and the Blavatnik National Award in Chemistry (2021).
Eliminating the use of critical transition metals in Li-ion battery cathodes is a key challenge for electrifying the transportation sector, especially in consumer vehicles. Vast resources are expended for replacing cobalt or minimizing the nickel content in commercial batteries, yet all rely on controlled doping of other elements (e.g. Mo, Mg, Ti, Mn, Nb) into ternary oxides to balance energy, power, safety, and cycling lives of such cathodes. An alternative strategy is to completely move away from inorganic oxides and use redox active organic materials, composed of just carbon, nitrogen, and oxygen, as cathodes. Despite the longstanding interest in developing organic cathodes, they generally perform poorly under practical conditions due to their relatively high solubility in battery electrolytes, which saps their cycle life, and low intrinsic electrical conductivity, which imposes needs for large amounts of additives and binders.
Here, we will describe the use of TAQ, a fused organic molecule with a layered structure that enables high electrical conductivity and complete insolubility in electrolytes, as a practically competitive cathode for Li-ion batteries. TAQ, made from just C, N, O, and H has higher energy density than incumbent commercial metal oxides at any charge-discharge rate, and maintains high capacity over thousands of cycles. It can deliver high charge capacity through reversible Li intercalation/de-intercalation between 2D molecular layers held together by a strong lattice of hydrogen bonds. Optimized electrodes made from TAQ outperform all commercial cathodes (including abundant lithium iron phosphate) at all charge-discharge rates, while retaining high capacity over thousands of cycles. Beyond the relevant battery metrics themselves, the structural and compositional principles underlining the electrochemical performance of TAQ provide a blueprint for the design and use of organic electrode materials in practical batteries.
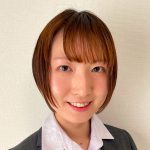
Misae Otoyama
Senior Reseracher
National Institute of Advanced Industrial Science and Technology (AIST)
Japan
Dr. Misae Otoyama is a senior researcher in the Department of Energy and Environment at National Institute of Advanced Industrial Science and Technology (AIST). She joined Advanced Electrochemical Device Research Group at AIST in 2020. Her research interests include materials chemistry and electrochemistry with a particular emphasis on development and characterization of all-solid-state batteries and solid electrolytes. She is a member of the national research projects for next-generation batteries: ALCA-SPRING (2020–2023), SOLiD-EV (2021–2023), and GteX (2023–present).
When she was a doctoral student, she joined the Prof. Jean-Marie Tarascon’s lab at the Collège de France as a visiting researcher and studied on synthesis and electrochemical activity of Na(Li)-rich ruthenium oxides (2017–2018). She received her Ph.D. degree in engineering from Osaka Prefecture University with her doctoral thesis entitled “Reaction Analyses of Composite Electrodes for Designing High Performance All-Solid-State Lithium Batteries” in 2020.
Among her several awards, she was the youngest researcher to receive the award from the committee of battery technology at the 60th battery symposium in Japan (2019). She was also the recipient of the 10th Japan Society for the Promotion of Science (JSPS) Ikushi Prize as an outstanding doctoral student who can be expected to contribute to Japan’s future scientific advancement (2020).
All-solid-state lithium sulfur batteries are attracted much attention as promising next-generation energy storage devices because of their high theoretical energy density. Metal polysulfide positive electrodes LixVSy (x = 5–9, y = 4–6) were synthesized by mechanochemical treatment of Li2S and V2S3. XRD, XAFS and TEM measurements revealed that LixVSy electrodes were consisted of nanocomposite of Li2S and LiVS2. Although Li2S is an insulator, LixVSy electrodes show high electronic conductivity of 10−1–10−2 S cm−1 because they contain LiVS2 with a high electronic conductivity. All-solid-state cells with composite positive electrodes of Li8VS5.5 and solid electrolytes at 80:20 (wt%) showed over 740 mAh g−1, which was 98% of the theoretical capacity. The cells with LixVSy need no conductive additives because of its high electronic conductivity. From the results of XRD and XAFS measurements of LixVSy electrodes before and after charge-discharge tests, delithiation and lithiation proceeded reversibly at Li2S and LiVS2. DSC measurements exhibited total heat of LixVSy was smaller than that of NCM electrodes. LixVSy is a promising positive electrode for high energy all-solid-state batteries because it shows a high capacity with high loading and fine thermal stability.
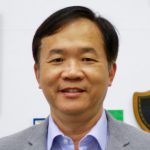
Nae-Lih Wu
Professor
National Taiwan University
Taiwan
Dr. Nae-Lih Wu is a tenured University Distinguished Professor at the Chemical Engineering Department, National Taiwan University, and serving as associate editor of the Electrochemical Society journals. His research interests include (1) electrochemical energy storage devices, including supercapacitors and rechargeable batteries; (2) nano-materials synthesis and applications; and (3) advanced synchrotron X-ray spectroscopy analyses for energy materials. His current research on Li-ion batteries focuses on active material interface engineering for fast charging and enhanced safety and the development of all-solid-state Li-ion batteries.
All-solid-state lithium-ion batteries (ASSLIBs) have been considered suitable alternatives to commercial lithium-ion batteries (LIBs) in the aspect of safety issues that come from the use of inflammable solid electrolytes to replace organic flammable electrolytes. Nevertheless, there remains much room for a better understanding of their properties and behaviors to upgrade their performance to reach the practical application level. Ni-rich layered oxides, LiNi1-x-yCoxMnyO2 (NCM), are promising cathodes for high-energy ASSLIBs because of their high capacities and redox potentials, and low material cost when compared with conventional LiCoO2. However, certain challenges associated with their use in ASSLIBs must be addressed for their effective use and industrialization. In particular, the structural integrity of the all-solid-state NCM composite electrodes suffers from not only intragranular cracks but also debonding at the cathode-solid electrolyte (SE) interface. The latter constitutes the major cause of fast capacity fade. To overcome this problem, it is a common practice to apply very high stacking pressure, typically higher than several tens MPa, on the ASSLIBs during operation. Lowering the battery operation stacking pressure, for example, to the level of the liquid-electrolyte batteries will certainly facilitate the wider applications of ASSLIBs. Using the composite cathode consisting of a LiNi0.83Co0.12Mn0.05O2 (NCM811) cathode and brittle Li3InCl6 (LIC) solid electrolyte (SE), this study demonstrates that forming a chemically fused cathode-solid electrolyte interface via limited interfacial reactions may serve as an effective means to mitigate interface debonding so as to enable ASSLIBs to maintain long-term operation under reduced stacking pressures.
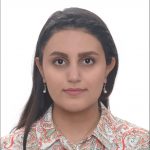
Nafiseh Zaker
Research Engineer
Natural Resources Canada | CanmetMATERIALS
Canada
With a Ph.D. in Material Science and Engineering from McMaster University, Dr. Nafiseh Zaker’s research focused on investigating the modification effects of coating, core-shell structures, and dopant introductions in Ni-rich cathode materials. Through advanced electron and synchrotron-based techniques, she explored the microstructural influences of these modification methods, aiming to correlate microstructure with battery performance. Joining CanmetMATERIALS laboratory in Hamilton in 2023, she expanded her focus beyond the characterization of different alloys to delve into critical minerals. Particularly, Nafiseh continued her research on Co-free cathodes alongside characterizing recycled graphite from Black Mass, crucial for advancing sustainable and green energy solutions.
Sustainability for lithium-ion batteries (LIBs) is driven by the necessity to reduce reliance on cobalt, a critical mineral entangled with environmental and ethical concerns, while also addressing the challenge of graphite resource depletion. This presentation highlights advancements in photon- and electron-based characterization strategies aimed at enhancing LIBs ecological balance.
First, we explore the enrichment of polycrystalline nickel-rich cathode structures with tungsten (W), unveiling the role of W in improving their electrochemical performance and structural stability. Employing advanced synchrotron-based characterization and electron microscopy techniques, discover various W-rich phases in both amorphous and crystalline forms with heterogeneity distribution, contributing to better cycling performance.
Next, we showcase the innovative application of W as a dopant in all-dry single crystal cathodes, highlighting the advancement in using single crystal and attempt to control over cathode structure and composition by this approach. High-resolution electron microscopy explains structural details about the distribution of transmission metals and introduced W elements, illustrating their potential to revolutionize LIB performance by enhancing energy density and cycling stability.
Furthermore, we present a comprehensive study on the recycling and purifying graphite for anodes from black mass, a byproduct of LIB recycling processes. Employing advanced electron microscopy techniques along with other chemical and electrochemical analyses, we pinpoint key parameters critical for evaluating the cycling performance of recycled graphite, paving the way for the development of sustainable anode materials with minimal environmental impact.
These advancements could help facilitating the widespread adoption of clean energy technologies and reducing carbon emissions while diminishing economic dependency on imported virgin materials.
Keywords: Electron microscopy, Sustainability, Cathode structures, Graphite reclamation, Tungsten doping, Recycling and Lithium-ion batteries.
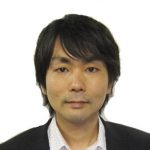
Naoaki Yabuuchi
Professor
Yokohama National University
Japan
Naoaki Yabuuchi is a professor at Yokohama National University. He completed his PhD at Osaka City University in 2006 and his postdoc at MIT, with research expertise in the development of new electrode materials and study on reaction mechanisms for high energy Li/Na batteries. He has over 130 publications in these areas. He is a fellow of the Royal Society of Chemistry and an associated editor of Energy Storage Materials. He is the recipient of First International Award, “Science Award Electrochemistry” by Volkswagen and BASF, The 2nd ISSI Young Scientist Award, and ISE Prize for Applied Electrochemistry, The Periodic Table of Younger Chemists (Niobium) from The International Union of Pure and Applied Chemistry (IUPAC) among other honors.
Ni-enriched layered materials are used as electrode materials of Li-ion batteries for electric vehicle applications. Stoichiometric LiNiO2 with cationic Ni3+/Ni4+ redox is the ideal electrode material, but the gradual loss of capacity at the high voltage region, associated with Ni ion migration, hinders its use for practical applications.1 Recently, the importance of non-stoichiometry and anti-site defects is discussed for LiNiO2, and highly reversible pure Ni-based layered materials without metal substitution is successfully developed through defects engineering.1
Another important target is the development of a practical and high-energy Co-/Ni-free Mn-based positive electrode material, which is necessary for the economical electric vehicles. Nanostructured Mn-based electrode materials are proposed as emerging materials for this purpose.2 However, these materials are generally synthesized by high-energy milling, which cannot be adopted for mass production. Recently, nanostructured LiMnO2 with high-energy density (~800 Wh kg–1) is successfully synthesized by using a conventional calcination reaction, which is potentially used for economical electric vehicle applications.3
To develop safe and high-energy Li-ion batteries, the use of solid electrolyte is an important strategy. Nevertheless, inevitable volume changes of electrode materials on cycling lead to the difficulty to maintain the stable interface between electrode materials and electrolyte. Recently, a nanostructured cation-disordered rocksalt oxide with a dimensionally invariable character is developed. Indeed, the excellent reversibility with solid electrolyte is achieved.5
From these results, the importance of nano-structured lithium insertion materials for practical Li-ion battery applications is discussed.
References
- Konuma et al., and N. Yabuuchi, Energy Storage Materials, 66, 103200 (2024).
- Kanno et al., and N. Yabuuchi, ACS Energy Letters, 8, 2753 (2023).
- Miyaoka et al., and N. Yabuuchi, submitted
- R. Fukuma et al., and N. Yabuuchi, ACS Central Science, 8, 775 (2022).
- I. Konuma et al., and N. Yabuuchi, Nature Materials, 22, 225 (2023).
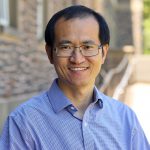
Penghao Xiao
Assistant Professor
Dalhousie University
Canada
Dr. Penghao Xiao is an assistant professor in the Department of Physics and Atmospheric Science at Dalhousie University in Halifax, NS Canada. His research focuses on simulation of materials from first principles, with a particular interest on the atomistic kinetic processes that dictates materials’ synthesis, performance, and degradation. Dr. Xiao obtained his Ph.D. from the University of Texas at Austin in 2014. He did his postdoc trainings at Lawrence Berkeley National Laboratory and then at Lawrence Livermore National Laboratory in California before joining Dalhousie in 2021.
High-Ni layered oxide cathodes have garnered much attention due to their balance between high capacity, low cost, and long cycle life. However, these high-Ni oxides exhibit profound capacity loss. In the first cycle, a notable portion of Li cannot be inserted back at the end of discharge. With continued cycling, an escalating portion of Li cannot be exacted at the end of charge, up to 25% of the theoretical capacity. These capacity losses erode the advantages offered by high-Ni oxides and impede their practical application. There is a lack of consensus on the underlying mechanisms, particularly the asymmetry between charge and discharge.
The intercalation of Li in cathode materials involves several kinetic processes, including diffusion in inhomogeneous media, phase nucleation/transition, and surface charge transfer. These processes are often coupled with each other, posting challenges in pinpointing the rate-limiting step. Here, we introduce a first-principles-based atomistic simulation framework to integrate these processes without empirical parameters, aiming to uncover the evolving rate-limiting step during charge/discharge without preconceived assumptions.
Using LiNiO2 as a mode system, our simulations successfully reproduce the first-cycle irreversible capacity loss at the end of discharge. At the end of charge, the rate-limiting step switches from diffusion to the H2-H3 phase transition. After cycling, the formation of a surface rock-salt-like phase suppresses the nucleation of the H3 phase near the surface, significantly slowing down its formation. Even prior to capacity loss, a low coverage of the surface densified phase exacerbates the asymmetry between charge and discharge at the H2-H3 plateau by only hindering charge kinetics. Furthermore, our simulations indicate that the densified phase has minimum impact on charge/discharge behavior for intermediate Li content. These findings align well with various experimental observations, underscoring the utility of our simulation framework in elucidating the complex interplay of kinetic processes in battery materials.
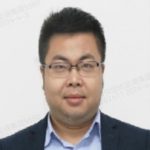
Pan Yi
Head of Basic Research Team
BYD Co.
China
Worked for the lithium-ion battery business of BYD Co. for 16 years, engaged in the development of new energy vehicle battery technology. Has been responsible for various technology research and product development projects. Currently serves as the head of the basic research team.
This study focuses on fast charging of lithium-ion batteries for electric vehicles (EVs), identifying disparate current density and state of charge (SOC) uniformity across different dimensions as critical constraints. Key control variables encompass electrode thickness, electrode structure, and temperature field distribution. Blade cells exhibit significant advantages in fast charging.
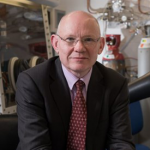
Professor Sir Peter Bruce, FRS
Wolfson Professor of Materials
University of Oxford
United Kingdom
Professor Sir Peter Bruce FRS is the Wolfson Professor of Materials at the University of Oxford and Chief Scientist of the Faraday Institution.
Peter’s research interests embrace materials chemistry and electrochemistry, with a particular emphasis on energy storage, especially lithium and sodium batteries. Recent efforts have focused on revealing the fundamental mechanisms occurring in solid-state batteries and developing strategies to mitigate issues at the interfaces, understanding anomalous oxygen redox in high capacity Li-ion cathodes, and the challenges of the lithium-air battery.
Peter’s research has been recognised with a number of prestigious awards and fellowships, including from the Royal Society of Chemistry, the Electrochemical Society and the German Chemical Society. He has been named as a Highly Cited Researcher by Thomson Reuters/Clarivate every year since 2015. Between 2018 and 2023 he served as the Physical Secretary and Vice-President of the Royal Society (UK’s national science academy). In the 2022 Birthday Honours List, Peter received a knighthood for his services to science and innovation.
Solid-state batteries based on a ceramic electrolyte and lithium metal anode have the potential to improve battery safety and energy density, but both charging and discharging rates are limited to below what is required. On discharge, voids form in the lithium metal at the Li/solid electrolyte interface due to limited Li metal creep at practical stack pressures and under practical current densities. These voids accumulate on cycling, leading to detachment of the Li anode and consequently high local currents during charge, triggering the growth of dendrites (filaments of Li metal that penetrate the ceramic electrolyte) resulting in short-circuit and cell failure.
Furthermore, even without any prior voiding, charging currents are limited to levels too low for practical applications by dendrite formation. Important efforts are being made to understand dendrites in ceramic electrolytes and to mitigate them.
We describe the formation and progression of lithium dendrites, informed by observing dendritic cracks operando using X-ray computed tomography (XCT). Dendrites are found to follow a two-stage process of initiation then propagation, with distinct mechanisms for each. Initiation occurs in sub-surface pores where pressure builds to exceed the local fracture strength at the grain boundaries, by the mechanism of slow lithium extrusion. Propagation involves dry cracks, with Li driving the crack forward from the rear by a wedge-opening mechanism, rather than lithium at the crack tip, as had often been assumed previously. Informed by the description of dendrite cracks, we investigated the relationship between the microstructure of the solid electrolyte and the critical current at and above which dendrites form. We show that by controlling the microstructure of the Argyrodite (Li6PS5Cl) solid electrolyte the critical current for dendrite formation can be determined. We also discuss the effect of contouring or roughening the lithium/solid electrolyte interface and the extent to which doing so can increase the critical current for dendrites.
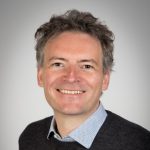
Philip Adelhelm
Professor of Physical Chemistry and Electrochemistry
Humboldt-University
Germany
Philipp Adelhelm is Professor of Physical Chemistry and Electrochemistry at Humboldt-University Berlin, Germany, and has been working on inorganic electrode materials for sodium-ion batteries since 2010. Currently, his main interests are layered materials (oxides, sulfides, graphite) and metals, and operando/in-situ tools such as optical microscopy, dilatometry, DEMS, and synchrotron methods. He is co-editor of the book “Sodium-Ion Batteries: Materials, Characterization, and Technology” (Wiley-VCH, 2022, Titirici/Adelhelm/Hu) and leads a joint research group on operando battery analysis between Humboldt-University Berlin and Helmholtz-Zentrum Berlin (HZB).
Layered materials are the foundation of modern Li-ion battery technology and are also used in the Na-ion batteries currently entering the market.[1],[2] A very successful strategy for tuning the properties of layered oxides is to change their composition, e.g. moving from LiCoO2 to NMC or NCA chemistries. This approach, along with understanding the redox activity of oxygen, is also currently a hot topic for Na-ion batteries too. Understanding the effects of doping / chemical substitution of layered oxides is complicated and requires a variety of analytical tools including synchrotron-based methods as well as theory, for example[3],[4]. A second, much less explored strategy for tuning the properties of layered materials is the co-intercalation of solvent molecules. An excellent example is the intercalation of solvated Na-ions into graphite, leading to the family of so-called ternary (or quaternary) graphite intercalation compounds. The use of co-intercalation in electrode reactions opens up a very diverse field of research, but at the same time there are also challenges that can be studied using, for example, operando electrochemical microscopy and operando electrochemical dilatometry. The talk will also present a new model for the formation of these compounds, which is very different from previous assumptions.[5] Recently, the concept of solvent co-intercalation has also been demonstrated for a layered sulfide, suggesting that also cathode materials may be tuned by co-intercalation.[6]
[1] Sodium-ion batteries: Materials, Characterization and Technology ISBN: 978-3-527-34709-4, Wiley Dec 2022, Titirici/Adelhelm/Hu (Editors)
[2] P. Nayak et al. Angew. Chemie. Int. Ed., 2018, DOI: 10.1002/anie.201703772
[3] L. Yang et al. Adv. Functional Materials, 2021, DOI: 10.1002/adfm.202102939
[4] Y. Li et al., Adv. Materials 2024, DOI: 10.1002/adma.202309842
[5] G. Avall et al. Adv. Energy Materials, 2023, DOI: 10.1002/aenm.202301944
[6] G. Ferrero et al. Adv. Energy Materials, 2022, DOI: 10.1002/aenm.202202377
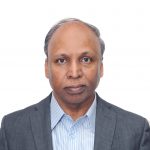
Palani Balaya
Associate Professor
National University of Singapore
Singapore
Before moving to National University of Singapore, Dr. Palani Balaya worked as a Guest Scientist (2001-2006) at Max Planck Institute for Solid State Research, Stuttgart in the area of Nano-ionics. He joined Department of Mechanical Engineering at College of Design and Engineering, NUS as an Assistant Professor in 2007. Since 2014, he works as an Associate Professor at NUS. His research area includes developing safe high-power lithium-ion battery and sodium-ion battery. He served as a Topical (Battery, Fuel Cell, Capacitor) Editor for Journal of Solid-State Electrochemistry (2013-2017). Since 2022, serving as Associate Editor for the International Journal of Applied Ceramic Technology (Wiley), Editorial Board Member of Ceramics International (Elsevier, since 2022) and for journal, Batteries (MDPI, since 2022). Elected as an Academician by the World Academy of Ceramics, Italy (2019) and elected as a Fellow of the American Ceramic Society (ACerS) in 2019. Recognized as an ACerS Global Ambassador in 2016, recipient of Global Star Award (2015) from ACerS, Late Shri Har Mahandar Singh Chhatwal Memorial Award (2015) from Indian Ceramic Society (ICS), M.G. Bhagat Memorial Lecture Award (2021) from ICS, etc. He served in Inter-governmental Panel on Climate Change as a Co-ordinating Lead Author for preparing a Special Report on Renewable Energy Sources and Climate Change Mitigation, in 2011. He served as the Chair of the Engineering Ceramic Division of ACerS (2022-23). Delivered more than 150 talks (Plenary/Keynote/Invited) at international conferences/meetings. Published 114 articles with h-index of 48 and citations of ~10500 (GS).
Recent fire incidents involving state-of-the-art Li-ion battery (LIB) systems underscore the imperative to prioritize safety improvements. While precise cause of thermal runaway still remains debatable, internal shorting within the cells when subjected to electrical, thermal and mechanical stresses is indeed a key factor.
This presentation delves into the critical issue of excess heat generation in LIBs. We report our findings on two commonly employed Li-ion cells using oxide-based cathodes (Ni-rich NMC or NCA cells) and carbon-coated LiFePO4 cathode (LFP cells) with graphite anode. Notably, our investigation reveals a significant correlation between heat generation and internal resistance. Among various contributions to internal resistance, authors show that the charge transfer resistance as well as Li diffusion at the cathodes seem to be the major sources causing unwanted Joule heating.
This talk will address various means of reducing internal resistance across different cell chemistries thus minimizing heat generation to assure safe operation of Li-ion cells especially at high rates. The following case studies will be presented, demonstrating their effectiveness in improving both performance and safety:
- Integrating nano-size LFP along with carbon coating improves charge transfer reaction in LFP cells as compared to micron size oxide-based cathodes.
- Utilizing a controlled synthesis technique to create nanostructured mesoporous LFP increases anti-site defect concentration. This Li-Fe defect enhances the 2 – D Li diffusion by more than an order of magnitude which helps to suppress voltage polarization substantially at high C rates.
- Doping Mg at Fe site in LiMnFePO4 (LMFP) solid solution effectively helps in improving Li diffusion which results in reduced heat generation and enhanced storage performance.
Full cell data using LMFP vs titanate based anode materials will be presented to further emphasize their potential for enhancing Li-ion battery safety.
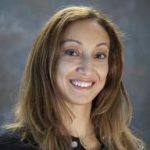
Rana Mohtadi
Senior Principal Scientist
Toyota Research Institute of North America
USA
Dr. Rana Mohtadi is a Senior Principal Scientist at the materials research department at Toyota Research Institute of North America TRINA and has been in the decarbonization R&D field for over two decades. She has been spearheading and leading research for the creation, design and demonstration of transformative materials for chemical and electrochemical energy storage technologies, including the research of novel battery electrolyte chemistries for liquid and solid state batteries, and has contributed important advancements in these areas. She has published influential research and has been awarded over 30 patents. Notable recognitions include receiving the R&D100 award (2011) and being named in “40 under 40” by the Automotive News (2014) and Crain’s Detroit Business (2014). She was recognized and showcased in the “We Run on Brain Power” initiative by the State Governor in 2015 as a face of innovation in the State of Michigan, USA. More recently, she was elected to serve as Chair for the Metal-Hydrogen Systems Gordon Research Conference (2025). Dr. Mohtadi also acts as a Principal Investigator at the Advanced Institute for Materials Research AIMR at Tohoku University in Japan (Researcher | AIMR (tohoku.ac.jp)).
- Materials Research Department, Toyota Research Institute of North America, Ann Arbor MI 48105, USA
- Advanced Institute for Materials Research (AIMR), Tohoku University, Sendai, Japan
Beyond Li-ion batteries have the potential to forge a path forward toward enabling large scale implementation of electrochemical energy storage systems. In particular technologies like solid state battery technologies and those incorporating metallic anodes have lured an ever expanding R&D global efforts motivated by promises of increased energy density and enhanced overall performances. However, enabling systems that are optimal remain a challenge. One important key to achieving the desired performances lies in the electrolytes. Herein, I will address issues facing beyond Li-ion batteries and explain the advancements made in our laboratories.
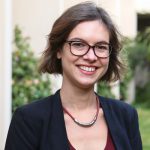
Raphaële Clément
Assistant Professor
University of California Santa Barbara
USA
Raphaële Clément is an Assistant Professor in the Materials Department at the University of California Santa Barbara since 2018. She received her Ph.D. in Chemistry in 2016 from the University of Cambridge, working under the supervision of Prof. Dame Clare Grey. She then joined the group of Prof. Gerbrand Ceder as a postdoc at the University of California Berkeley. At UCSB, the Clément group is interested in establishing materials design rules, and in optimizing materials processing approaches to advance electrochemical energy storage. The group’s expertise lies in the development and deployment of magnetic resonance techniques for the study of battery materials and beyond, with a strong emphasis on operando tools. She received an NSF CAREER award in 2022, the Materials Today Rising Star Award and the ISE Prize in Electrochemical Materials Science from the International Society of Electrochemistry in 2023, and a 2024 Camille Dreyfus Teacher-Scholar Award. She is a Topical Editor for ACS Energy Letters.
An atomic-level understanding of the links between structure, including polymorphism, defects, and disorder, on the ease and reversibility of ion intercalation is key to accelerating the development of next-generation cathodes. We do so by combining operando/in situ/ex situ characterization, and simulations. Starting with LiNiO2, we have determined the concentration of planar defects in the as-synthesized material and quantified their contribution to the first cycle irreversibility. We have also identified, for the first time, the composition of the bulk “fatigued” (i.e., partially electrochemically active) phases formed during long-term cycling. When it comes to disordered rock salt oxide (DRX) cathodes, we have developed methods to assess the amount of F incorporated into the bulk structure. These methods have allowed us to optimize the synthesis of DRX via standard solid-state methods and microwave heating, and show that, at a fixed F content, increasing the Mn content results in greater capacity and capacity retention. Finally, I will discuss our recent findings on the partial “δ phase” transformation taking place in Mn-rich DRX during electrochemical cycling. By systematically studying δ phase formation in Mn-rich and Mn-poor DRX compounds subjected to chemical delithiation and a heat treatment, we have found a larger driving force and a lower activation energy towards local cation rearrangements in Mn-rich DRX compounds, with DRX and spinel lattices matching and forming a continuous and periodic framework without clear boundaries.
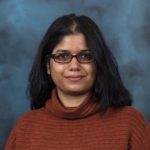
Ritu Sahore
R&D Associate
Oak Ridge National Laboratory
USA
Ritu Sahore is a R&D Associate in the Energy Storage and Conversion group within the Chemical Sciences Division at Oak Ridge National Laboratory, since 2021. She received her Ph.D. in Materials Science and Engineering from Cornell University in 2015, followed by two postdoctoral appointments at Argonne National Laboratory (2015-2018) and Oak Ridge National Laboratory (2018-2021). She has over 10 years of research experience in the field of batteries with chemistries ranging from lithium-sulfur, lithium-ion, and solid-state batteries, with over 35 peer-reviewed publications. She enjoys looking at the battery chemistries at a cell level and exploring the various cell components’ interactions. Her current research includes understanding cell degradation mechanisms in high-voltage lithium-ion and polymer electrolytes-based solid-state battery chemistries, as well as exploring the fundamental electrochemical properties of polymer-ceramic composite electrolytes.
Cycling of NMC-based lithium-ion batteries to high cut-off voltages leads to accelerated degradation at the NMC cathode surface, primarily, oxygen loss from the lattice which causes surface restructuring of the lattice from layered to rock-salt/spinel phases with very high impedance for Li-ion transport. Dissolution of transition-metals (TMs) also occurs with high-voltage cycling, whose migration and redeposition at the graphite anode causes accelerated lithium inventory loss due to reductive side reactions, and generate preferential sites for lithium plating down the line. Several other phenomena get accelerated as well, such as generation of acidic protons and gaseous/soluble decomposition species due to solvent and salt decomposition.
However, a complete mechanistic understanding of the how these phenomena (O-loss, acid generation, other ligands generation) impact the TM dissolution is lacking. For example, it is unclear whether the dissolution can occur in the charged state when the metals are in their highest oxidization state, especially because the dissolved metal species typically detected in the cycled electrolyte are in lower oxidation states (2+, 3+). In this study, this question was targeted by aging graphite//NMC cells via long potentiostatic holds at UCV with/without discharge steps, and recording the metals deposited at the graphite anode after aging. While significant metal dissolution occurred with just potentiostatic holds, interestingly, a much greater amount is deposited at the anode when discharge steps are also included. Given that single crystal cathodes are used in this study which do not have the issue of intergranular cracking that is present in polycrystalline cathodes, this observation suggests a greater propensity of metal dissolution during the discharged state, or presence of intragranular cracking in single-crystal cathodes (as well) that would expose fresh cathode surface during discharge/charge cycling. Atomic structural changes from TM dissolution at the cathode surface in the charged/discharged state will also be presented.
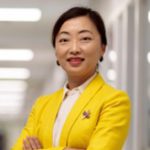
Shirley Meng
Professor
University of Chicago
USA
Dr. Y. Shirley Meng is a Professor at the Pritzker School of Molecular Engineering at the University of Chicago. She serves as the Chief Scientist of the Argonne Collaborative Center for Energy Storage Science (ACCESS) Argonne National Laboratory.
In this talk, I will discuss a few new perspectives about how materials innovation and interfacial science can accelerate the innovation of next generation energy storage materials and architectures, with a particular focus on anode-free all solid state batteries. Anode-free batteries possess the optimal cell architecture due to their reduced weight, volume, and cost. However, their implementation has been limited by unstable anode morphological changes and anode-liquid electrolyte interface reactions. An electrochemically stable solid electrolyte can solve these issues by enabling the deposition of dense sodium metal. Furthermore, a novel type of aluminum current collector can achieve intimate solid-solid contact with the solid electrolyte which allows highly reversible sodium plating and stripping at both high areal capacities and current densities, previously unobtainable with conventional aluminum foil. A sodium anode-free
all-solid-state battery full-cell is demonstrated with stable cycling for several hundred cycles. This cell architecture serves as a future direction for other battery chemistries to enable low-cost, high- energy-density, and fast charging batteries.
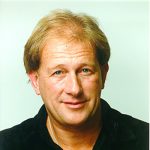
Steven Visco
CEO
PolyPlus
Steven Visco is the Chief Executive Officer, CTO, and founder of PolyPlus Battery Company in Berkeley, California, as well as a Guest Scientist in the Materials Science Division at the Lawrence Berkeley National Laboratory. Steven J. Visco currently holds 150 U.S. patents, more than 200 international patents and has authored over 80 journal articles, as well as books, monographs and other publications. Dr. Visco graduated with a B.S. in Chemistry from the University of Massachusetts in 1977 and received his Ph.D. in Physical Chemistry from Brown University in 1982. Dr. Visco then joined the staff at the Lawrence Berkeley National Laboratory as a Principal Investigator in the Materials Sciences Division in 1984 where his research interests have included advanced batteries and fuel cells. Steven Visco co-founded PolyPlus Battery Company in 1991. In 2013 Dr. Visco was selected by the City of Berkeley for a “Visionary Award” for his work in next generation batteries. Steve also serves on the Technical Advisory the CIC Energigune Institute in Miñano, Spain and was awarded the 2011 International Battery Association Award for “Outstanding Contributions to the Development of Lithium-Air and Lithium-Water Batteries.” PolyPlus Battery Company was selected by TIME magazine for its 50 Best Inventions of 2011 Issue, and was selected for a Gold Edison Award in 2012. In May 2015 Dr. Visco was elected a Fellow of the Electrochemical Society.
PolyPlus Battery Company was originally founded to commercialize Li/S batteries and developed the ether-based electrolytes used in most, if not all, advanced Li/S chemistries. The presence of dissolved polysulfides which react with the negative electrode leads to parasitic cell reactions (polysulfide shuttle) and poor cycling, motived the company to develop “protected lithium electrodes.” Since that time the company has pioneered the use of solid electrolytes to chemically isolate the Li electrode while promoting reversible cycling. PolyPlus uses both polycrystalline and amorphous glasses to build protected electrodes for different applications. The ceramic electrolyte Li1+xAlxTi2–x(PO4)3 (LATP) has an ionic conductivity of greater than 3 x 10-4 S/cm and is remarkably stable to aqueous electrolytes; allowing PolyPlus to build primary Li/Air batteries that have achieved close to 1,000 Wh/kg in external testing, and Li/Water batteries which will soon be commercialized at energy densities of ~ 1500 Wh/kg and 1000 Wh/l (this is the highest specific energy ever demonstrated). PolyPlus is also working with sulfide glasses for rechargeable applications. We work with sulfide glasses having ionic conductivities of 10-3 S/cm, and with a sufficiently large window between the glass transition temperature and the crystallization temperature to process the glass into thin sheets. Since sulfide solid electrolytes are moisture sensitive, draw-down is done inside a draw tower that is enclosed in a vertical glovebox. We are presently developing 3D interconnected cathodes having no carbon additives and sufficient porosity to infiltrate sulfide solid electrolyte to be used in fully solid-state rechargeable Li metal batteries. The challenges and successes of these approaches will be addressed in the presentation.
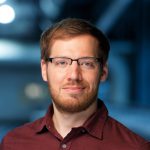
Toby Bond
Senior Scientist
Canadian Light Source
USA
Toby Bond is a Senior Scientist in the Industrial Science group at the Canadian Light Source (CLS), Canada’s national synchrotron facility. He is a specialist in x-ray imaging of energy storage devices, specializing in in-situ and operando analysis of batteries and fuel cells for industrial clients of the CLS. Toby is an electrochemist by training, having completed his B.Sc. in Chemistry at the University of Saskatchewan in 2010, followed by a M.Sc. in Chemistry at Dalhousie University in 2012, where he developed prototype high-precision coulometry instruments with Dr. Jeff Dahn. Toby has been with the CLS since 2014, and is currently finishing a Ph.D. in the Dahn lab while continuing to work at the CLS.
As adoption of EVs and e-mobility devices becomes more widespread, the development of cathodes with higher energy density has become a major focus of cell manufacturers and OEMs. Ni-rich NMC cathodes in particular have been widely used for these applications. However, these materials are known to experience significant mechanical stress during cycling as a result of large anisotropic volume expansion and contraction. After repeated cycling, these stresses can lead to microcracking of secondary particles, which has been well-characterized in the literature using post-mortem techniques such as electron microscopy. While powerful and informative, this kind of post-mortem analysis requires destructive sampling of the electrode and provides only a limited understanding of how microcracking affects the operation and multi-scale structure of the cell.
Using synchrotron-based operando X-ray diffraction and microscopy techniques, we have characterized significant microstructural and kinetic changes in NMC622/graphite pouch cells that have been cycled for over two years. These include dramatic changes in porosity, particle morphology, electrode thickness, electrolyte distribution, and spatial distribution of lithiation state during cycling. Using non-destructive, time-resolved measurements of unmodified cells, the progression of microcracking can be tracked over months of cycling, allowing us to explore its effects at the particle, electrode, and cell level. The ability to characterize and accurately model this type of degradation has increasingly important for high-cycle-count applications like vehicle-to-grid energy storage and second-life batteries.
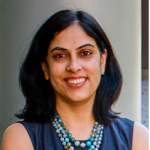
Vibha Kalra
Fred H. Rhodes Professor of Chemical Engineering
Cornell University
USA
Dr. Vibha Kalra is Fred H. Rhodes Professor in the Smith School of Chemical and Biomolecular Engineering at Cornell University. She also serves as an editor of Chemical Engineering Science journal, since 2013. Prior to joining Cornell University in January 2024, Kalra was George B. Francis professor in the Department of Chemical and Biological Engineering at Drexel University, starting as an assistant professor in 2010. Kalra received her BS from the Indian Institute of Technology (IIT), Delhi, India in 2004 and PhD from Cornell University in 2009, both in Chemical Engineering. Her research group combines material assembly & characterization, study of fundamental electrochemical behavior, in-situ spectro-electrochemistry, and device assembly and testing to develop energy storage devices, including next-generation batteries and supercapacitors. She has published over 65 peer-reviewed journal articles and has 13 pending/issued patents in the field of energy storage. Kalra is a recipient of several awards including the NSF CAREER award (2012), ONR summer faculty fellowship award (2013), AIChE DVS Outstanding Faculty of the Year Award (2015), CBE Outstanding Service Award (2018), College of Engineering Outstanding Research Award, both at assistant professor level (2015) and at mid-career level (2020)), Provost Award for Outstanding Mid-Career Research Achievement (2020), and the COE Outstanding Innovation Award (2021). Kalra was also selected as a fellow of the Executive Leadership in Academic Technology, Engineering and Science (ELATES) program in 2022-23.
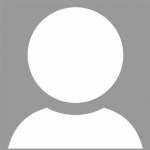
Wang Fang
Chief Scientist
China Automotive Technology & Research Center (CATARC)
China
Wang Fang, Ph.D., Chief scientist of China Automotive Technology & Research Center (CATARC),Professor-Level Senior Engineer. She has committed to the test technology research on new energy vehicle and key components for long term, and has created a test team with international influence, research topics including performance, safety, cycle life testing and battery failure analysis, etc.She has published more than 100 academic papers, more than 50 authorized patents and 3 monographs.
Lithium-ion traction batteries have been widely used in the field of electric transportation, but their safety has always been a focus of attention in the industry and scientific community. This report introduces the testing and evaluation techniques and safety requirements of traction batteries based on the actual operating conditions and scenarios of new energy vehicles. Based on the typical failure modes and influencing factors of batteries, introduce the testing evaluation methods of electrical damage, thermal damage, and mechanical damage, and analyze the typical damage and failure mechanisms of traction batteries from the material and battery levels.
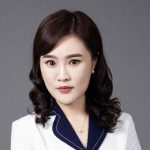
Yan Yu
Professor of Material Science
University of Science and Technology of China
China
Yan Yu is a full professor of material science at University of Science and Technology of China (USTC). She received her Ph.D. at USTC in 2006. From 2007 to 2008, she worked as a postdoctoral researcher at Florida International University. After that she received a Humboldt Research Fellowship and the Sofja Kovalevskaja award from the Alexander von Humboldt Foundation and worked at the Max Planck Institute for Solid-State Research in Stuttgart, Germany. Her current research interests mainly include the design of novel nanomaterials for clean energy, especially for batteries and the fundamental science of energy-storage systems. She was selected as a Thomson Reuters Highly Cited Researcher in the field of Materials Science. She also received several awards and honors, such as the National Science Fund for Distinguished Young Scholars, The 16th China Youth Science and Technology Award, Fellow of The Royal Society of Chemistry, etc.
Sodium batteries (SIBs) are of major importance, especially for large-scale energy storage devices, due to their low cost, abundant source of sodium and high safety. Designing and constructing advanced electrode materials and solid electrolyte materials are crucial to realize high-performance SIBs. For the cathode, we design several NASICON and Prussian blue cathode materials, and improving their electrical conductivity and structure stability by constructing hybrid structure and anion doping strategies. For the anode, to address the problems of Na metal anode in terms of unstable SEI structure, dendrite growth and huge volume expansion, we develop some effective strategies to regulate the interface of Na metal anodes: 1) designing 3D conductive skeleton to reduce local current density and induce uniform deposition of Na ions. 2) constructing artificial interface protective layer on the surface of Na metal anode to improve the mechanical toughness, enhance the ion diffusion ability and reduce the nucleation overpotential. 3) modifying the electrolyte and separator to induce uniform Na deposition and suppress the dendrite growth. Based on the above rational materials structure design, we obtain advanced SIBs with high energy density and long cycle life.
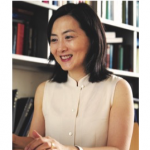
Yang Shao-Horn
Professor
MIT Department of Material Science and Engineering
USA
Yang Shao-Horn is JR East Professor of Engineering at Massachusetts Institute of Technology. Her research is centered on exploiting chemical/materials physics and physical/materials chemistry principles to understand and design charge transfer, kinetics and dynamics at the solid-gas and solid-liquid interfaces, critical to enable the deployment of clean air and clean energy technologies. Professor Shao-Horn is a scientist and entrepreneur in electrochemical science and engineering, among the top most cited female chemists in the world, focusing on clean energy solutions. She is a member of the National Academy of Engineering and a fellow of the American Association for the Advancement of Science, the Electrochemical Society, the National Academy of Inventors and the International Society of Electrochemistry.
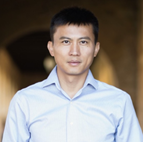
Yi Cui
Professor of Materials Science and Engineering
Stanford University
USA
At Stanford University, Yi Cui is Founding Director of Sustainability Accelerator, Fortinet Founders Professor of materials science and engineering, energy science and engineering, and of photon science at SLAC National Accelerator Laboratory. He earned his bachelor’s degree in chemistry in 1998 from the University of Science & Technology of China and his PhD in chemistry from Harvard University in 2002. Cui was a Miller Postdoctoral Fellow at the University of California, Berkeley from 2002 to 2005 before joining the Stanford faculty.
He works in the area of nanotechnology and clean energy technology. He is best-known for his works on reinventing batteries through nanotechnology. His introduction of silicon nanowires for lithium ion battery anodes in 2008 has launched a burst of nanostructure designs for batteries. He introduced additional silicon nanostructures, nanostructured sulfur cathodes, and Li metal anodes for high energy density batteries. He also invented new type of aqueous metal-hydrogen gas battery for grid scale energy storage with excellent power, safety, efficiency and durability. He pioneered the development of cryogenic electron microscopy technique for battery research and answer important long-standing questions in the field. His prolific and wide-ranging contributions (>560papers, >280,000 citations, H-index 265) solve important societal challenges while simultaneously seeking a deep scientific understanding of the underlying physical mechanisms.
He served as the Director of Stanford’s Precourt Institute for Energy (2021-023) and an Associate Editor of Nano Letters (2011-2023) and Co-director of the Battery 500 Consortium, Bay Area Photovoltaic Consortium, Stanford StorageX Initiative and Stanford’s Ecopreneurship program.
He is an elected member of the US National Academy of Sciences, fellow of the American Association for the Advancement of Science, fellow of the Materials Research Society, fellow of the Electrochemical Society, and fellow of the Royal Society of Chemistry. His selected honors include Global Energy Prize (2021), Ernest Orlando Lawrence Award (2021), Materials Research Society Medal (2020), Electrochemical Society Battery Technology Award (2019) and Blavatnik National Laureate (2017). He founded five companies to commercialize technologies from his lab and created economic values to United States: Amprius (NYSE: AMPX), 4C Air, EEnotech, LifeLabs Design, EnerVenue.
This talk presents a decade long research on the following topics related to Li metal anodes: 1) Designing host and interface materials to contain the volume fluctuation during plating and stripping; 2) Newly designed fluorinated electrolytes, suspension electrolytes and high entropy electrolytes for improving cycling coulombic efficiency; 3) Studying corrosion and capacity fading mechanisms leading to designing strategies for the recovery of isolated Li metal.
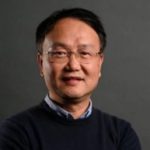
Yong Yang
Director
Xiamen University
China
Dr. Yong Yang is now a distinguished professor in Chemistry in the State Key Lab for Physical Chemistry of Solid Surface at Xiamen University since 1997. He now also serves as Editor for J Power Sources and the President of Internationa Battery Materials Associations(2024-2025). His major research interests include new Electrode/Electrolyte materials for Li/Na batteries, operando spectroscopic techniques and reaction mechanism study in electrochemical energy storage and conversion system. He has published 500+ papers in referred journals (H =91, Google Scholar) , 50+ patents and as leading editor of two books entitled “Solid State Electrochemistry” (Chinese, 2017) & “NMR/MRI of Electrochemical Energy Storage Materials & Devices” (English, 2021), He obtained several awards such as Outstanding Researchers in Fujian Province(2023), Technology award from Battery Division, ECS (2020), Distinguished Contribution Award from Chinese Electrochemical Society (2017) and Technology Award from International Battery Materials Association (2014). His main recent research interests are Solid-State batteries, interfacial electrochemical reaction mechanism and in-situ spectroscopic techniques such as NMR and XAS, and Li-ion Battery Ageing and Degradation Analysis for Power and Storage Applications.
All solid-state lithium-sulfur batteries (ASSLSBs) have attracted significant attention due to their enhanced safety and superior energy density. However, the considerable electrolyte decomposition and volume change during cycling poses some challenges, resulting in electrochemical-mechanical degradation. To address this issue, polypyrrole (PPy) is coated on a nitrogen-doped carbon nanotube (NCNT) network to exhibit suitable conductivity and compatibility with sulfide electrolyte. Functionally, PPy@NCNT provides a continuous and conductive pathway, reducing the tortuosity of charge transport. Mechanically, operando pressure measurements and atomic force microscopy (AFM) tests are conducted, demonstrating that the flexible and viscoelastic structure of PPy@NCNT can alleviate local stress. The axial pressure on the cathode can be reduced by 11% with a sulfur area capacity of 3 mg cm-2 at 30°C. At a high areal mass loading of 4.5 mg cm−2, a high areal capacity of 6 mAh cm−2 (1348 mAh g-1, 1C=1675 mA g-1) is achieved at 0.1C, maintaining stability over 300 cycles at 0.2C. Remarkably, it can achieve an areal capacity of over 8.8 mAh cm-2 and stable cycling even at 60°C. This study, being the first to regulate mechano-electrochemical action in ASSLSBs with flexible conducting polymer, highlighted the significance of integrating a continuous and elastic network into solid-state conversion-type cathodes. In addition, atomic layer deposition(ALD)-derived lithium phosphorus oxide is applied to the surface of carbon/sulfur particles to enhance the interfacial stability of the sulfur cathode and improve the electrochemical performance of ASSLSBs. The coating layer can inhibit electrolyte decomposition and improve interfacial stability by blocking electron conduction between carbon and electrolyte. Moreover, it not only serves as an ion-conducting layer to facilitate Li+ transport but also acts as a stress buffer layer to alleviate contact failure. The assembled ASSLSBs with sulfide electrolyte exhibit initial specific capacity of 1322 mAh g-1 at 0.2 C and capacity retention of 86.4% after 300 cycles. Furthermore, ASSLSBs maintain reversible capacity of 645 mAh g-1 at 0.5 A g-1 after 1000 cycles。 Finally,some new results about understanding of failure processes of Li metal anode in all-solid-state battery will be also presented.
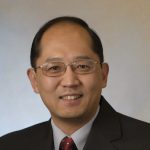
Yuan Gao
Lithium Americas Corporation
USA
A world renowned inventor, Dr Yuan Gao has over 30 years of experience in the complete supply chain of lithium-ion battery industry, from lithium extraction to batteries. One of his inventions, the Ti/Mg technology, enables high voltage lithium-ion batteries and is being practiced today in a vast majority of lithium-ion batteries for small portable electronics such as smart phones and tablets.
Dr Yuan Gao joined Pulead Technology in May 2014 and had served as its President and CEO until Sept 2019. Currently he is serving on the Board of Directors of Lithium Americas Corporation, as well as the Boards of Advisors of Nano One, Mitra Chem and Pure Lithium while advising Cobco, a cathode precursor producer in Morocco. Previously Dr Gao served as a Corporate Vice President at Molycorp (USA), and a Global Marketing Director and Technology Manager, as well as in other management positions at FMC Corporation Lithium Division, the predecessor to Livent (USA) and today Acardium Lithium.
Dr Gao received his BSc from the University of Science and Technology of China, and his PhD in Physics from the University of British Columbia in Canada. He received his postdoc training from Professor Jeff Dahn’s lab at Simon Fraser University. He has also received Executive Education from The Wharton Business School, University of Pennsylvania in the USA.
The average temperature on the surface of the earth has increased by 1.5 degree Celsius already compared to the pre-Industry time, which is believed a threshold for irreversible damages and a red line. Urgent actions are needed and electrification of transportation can go a long way in holding this line. This global challenge for all requires a global solution even in today’s deglobalizing world. While it makes perfect sense to develop parallel localized supply chains for supply security as well as local employment for improved sustainability, reinventing the wheel and rejecting decades of experience and know-how would significantly increase cost and delay the EV adaption. Also, past supply chains developed might be results of the then business eco system over there, and may not be applicable here and now. We will discuss those challenges and possible solutions.
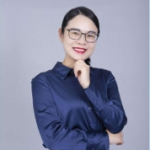
Yunxian Qian
CTO & General Manager of Battery Chemical BU
Shenzhen CAPCHEM Technology Co. Ltd.
China
Dr. Yunxian Qian: As the CTO and General Manager of Battery Chemical BU in Shenzhen Capchem Technology, Dr. Yunxian Qian has rich experience in material research and development in the field of lithium-ion batteries. During her tenure, she has received two national grants, published more than 20 articles in international top journals as the first author or corresponding author, with a total impact factor exceeding 150. She has applied for more than 100 invention patents as the first applicant, and led the establishment of more than 20 internal research and development projects in the company.
The modern lithium-ion battery (LIB) configuration was enabled by the “magic chemistry” between ethylene carbonate (EC) and graphitic carbon anode. Despite the constant changes of cathode chemistries with improved energy densities, EC-graphite combination remained static during the last three decades. Electrolytes in modern Li-ion batteries (LIBs) rely on additives of various structures to generate key interphasial chemistries needed for desired performances, although how these additives operate in battery environments remains little understood. In this work, different film-forming additives, including the state-of-art SEI and CEI additives were studied to investigate their influence on electrolyte-electrode interphase. Furthermore, the impact of electrolyte on the structural changes upon long-term cycling is further clarified. The correlation established among the additive structure, interphasial chemistries and cell performance offer the guide in designing the electrolytes with atomistic precision for future battery chemistries.
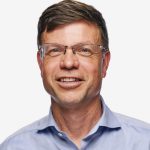
Kurt Kelty
Vice President
General Motors, Battery Cell & Pack (BCP)
USA
General Motors appointed Kurt as Vice President, Battery Cell & Pack (BCP) in February 2024. In this newly created role, he leads GM’s battery cell strategy and end-to-end battery approach, to include raw material usage, research, new technology development and investments, commercialization of cells and packs, and end of life opportunities.
Kurt is a globally recognized battery expert with more than 30 years of experience formulating and implementing energy storage technology in electronics, energy storage systems, and EVs – including work as a material supplier, cell-maker, and end user. He began his work in lithium-ion batteries in 1993 with Panasonic, where he created alliances and joint development programs to advance lithium-ion rechargeable batteries for portable applications. While at Panasonic, Kurt founded and led its battery research lab in Silicon Valley and created R&D alliances between Panasonic and other U.S. battery and fuel cell developers. Upon leaving Panasonic in 2006, Kurt joined Tesla and led Tesla’s battery cell development team for 11 years. At Tesla he was responsible for the technical exchanges and commercial negotiations with battery cell suppliers and early-stage battery cell developers. Kurt was a key driver in the creation of Tesla’s first Gigafactory, the largest lithium-ion battery and EV component factory in the world. Most recently, Kurt was a vice president at Sila, where he oversaw sales, business development, battery cell manufacturing partnerships and battery engineering involved in the adoption of Sila’s silicon anode material in EVs requiring high energy density and fast charge.
Kurt is a graduate of Swarthmore College with a bachelor’s degree in biology and a graduate of Stanford University, where he earned his MSc through Stanford Business School’s Sloan Program. Kurt is the author of 16 patents in the U.S. and Japan.
Kurt has also served as a Board Member for Auto Motive Power (sold to Ford) and an Advisory Board member to BP and other energy related companies.
GM has established a foundation to accelerate the investment in and development of battery technology with a robust supply chain to support its growth over the next decade. In this talk, Kurt will discuss GM’s strategies for investing in new technologies and how its in-house capabilities enhance those efforts, with an overview and rationale behind key investments made to date. He will also provide insights on the company’s approach and significant milestones moving forward.
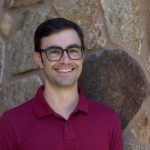
Tim Holme
Co-founder & Chief Technology Officer
QuantumScape
USA
Tim Holme got his BS in Physics and PhD in Mechanical Engineering from Stanford University. After co-founding QuantumScape in 2010, he has served as the CTO where he learns alongside a world-class team dedicated to the mission of making advanced batteries that enable long-range, high-performance, mass-market electric vehicles.
QuantumScape is developing a solid-state battery with a lithium-metal anode to enable long-range, faster charging, low-cost EVs. The technology features an anode that is lithium-free as manufactured, with the lithium being delivered entirely from the cathode material. The lithium-free approach offers a significant cost savings relative to approaches that utilize an excess lithium foil or vapor deposition process. This talk will highlight the scientific and engineering challenges in developing an anode-free solid-state battery, with a historical overview and a snapshot of the progress at QuantumScape. QuantumScape was founded in 2010 with a mission to revolutionize energy storage to enable a sustainable future.
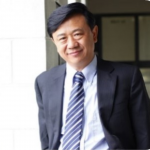
Andy Sun
Professor
University of Western Ontario
Canada
Dr. Xueliang (Andy) Sun is currently a Chair Professor at Eastern Institute of Technology (tentative), Ningbo, China, and an Adjunct Professor and Emeritus Distinguished University Professor at the University of Western Ontario, Canada. Dr. Sun is a Fellow of the Royal Society of Canada and a Fellow of Canadian Academy of Engineering and a Foreign Member of the Chinese Academy of Engineering. His research interests are focused on all-solid-state batteries and fuel cells. He is an Editor-in-Chief of “Electrochemical Energy Review” (IF=31) under Spring-Nature. Dr. Sun received his Ph.D degree in Materials Chemistry at the University of Manchester, UK, in 1999. Dr. Sun is a Vice Chairman of the International Academy of Electrochemical Energy Science (IAOEES). Dr. Sun is an author and co-author of over 660 refereed-journals with citations of over 70,000 times and H-index of 130. Dr. Sun was named as one of "Highly Cited Researchers". He edited 4 books and published 20 book chapters as well as filed 30 patents. He has given more than 200 plenary/keynote/invited talks in international conferences, symposia and workshops. Dr. Sun received various awards such as Professional Achievement Awards from Cross-cultural Professionals Association of Canada (CPAC, 2016), Award for Research Excellence in Materials Chemistry Winner from Canada Chemistry Society (2018), Award of Merit of the Federation of Chinese Canadian Professionals (2018), Western Hellmuth Prizes (The highest Research Achievement Award in the University, 2019), IBA Battery Technology Award (2021) and Distinguished University Professor (2023).
All-solid-state lithium metal batteries (ASSLMBs)1, 2 are pivotal for ensuring high safety, extended driving range, and rapid charging in electric vehicles. Despite these advantages, It is necessary to develop new solid-state electrolytes (SSEs) with thermodynamically stable to lithium metal to suppress lithium dendrite growth at high current densities and capacities exceeding 3 mAh/cm2. Recently, we developed a series of superionic conducting, highly lithium-compatible nitride SSEs, including vacancy-rich β-Li3N,3 vacancy-rich Li9N2Cl3,4 and antifluorite Li2.5N0.5S0.55 SSEs. These SSEs exhibit high room-temperature ionic conductivity up to 2.14×10-3 S/cm, surpassing most reported nitride-based SSEs, due to the high concentration of mobile Li ions and low Li ion hopping energy. Their thermodynamic stability with lithium metal enables the suppression of lithium dendrite growth even at high current densities (up to 45 mA/cm2) and capacities (up to 10 mAh/cm2). Additionally, nitride SSEs exhibit high stability in air, promising ease of industrial fabrication in battery dry rooms and practical applications for ASSLMBs. Coupled with halide-based electrolytes, ASSLMBs demonstrate high performance.
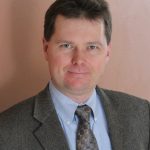
Borodin Oleg
DEVCOM Army Research Laboratory
USA
Oleg Borodin completed a Ph. D. in Chemical Engineering in 2000 at the University of Utah. He has been working on the molecular scale modeling of electrolytes, polymer solutions, polymer melts, composites, electrochemical interfaces, supercapacitors and electrolyte design publishing 240 articles in peer-reviewed journals and 6 book chapters. In 2010 Dr. Borodin joined DEVCOM Army Research Laboratory, where his research focused on the mechanisms of ionic transport, electrolyte stability, degradation and understanding of the electrochemical interfaces in lithium, sodium, zinc and magnesium batteries with aqueous and non-aqueous electrolytes utilizing quantum chemistry and molecular dynamics simulation methodologies. His is a Highly Cited Researcher according to Clarivate Analytics in the Cross-Field for 2021- 2023 and a recipient of a numerous awards including Army Research Laboratory Award for Science, UMD Invention of the Year, Army Civilian Service Commendation Medal.
A combination of (MD) molecular dynamics simulations and quantum chemistry calculations not only provide insight into the structure and ion transport mechanisms in lithium, sodium, magnesium, and zinc electrolytes but also guides electrolyte selection. I will focus on our recent findings of the molecular scale mechanisms leading to the improved aprotic electrolyte electrochemical stability on both lithium metal anodes and high voltage cathodes in lithium batteries, tailoring the solid electrolyte interphase (SEI) and cathode electrolyte interphase (CEI) composition to improve reversibility of cell cycling including improved low temperature performance. Next, I will discuss the transport mechanism in these electrolytes and its connections with a transference number extracted from MD simulations.